Українською
  In English
Feed aggregator
Shin-Etsu Chemical to develop QST substrate for 300mm GaN
🍁 Фестиваль «Японська осінь»
З 13 по 15 вересня на території Київського Політехнічного Інституту ім. Ігоря Сікорського відбудеться благодійний фестиваль «Японська осінь».
STPOWER Studio: 3 new topologies for accurate electro-thermal simulation on STPOWER devices
Author: STMicroelectronics
STPOWER Studio 4.0 just became available and now supports three new topologies (1-phase full bridge, 1-phase half-bridge, and 3-phase 3-level T-NPC) to cover significantly more applications. Previously, the online simulation tool only offered a 3-phase 2-level topology for motor drivers and photovoltaic inverters, which are some of the most popular use cases. Thanks to the robustness of the underlying architecture that ST recently updated and brought to the web, we are now able to build on top of the existing platform to make STPOWER Studio more versatile and assist more engineers in designing a more comprehensive range of power stages.
What is STPOWER Studio? A unique simulator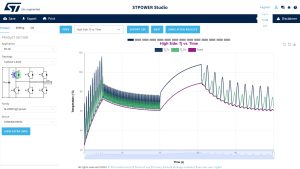
STPOWER Studio, a component of eDesignSuite, specializes in thermo-electrical simulations. As eDesignSuite transitioned to HTML5 to enhance its user interface, STPOWER Studio benefited from the same underlying architecture, enabling more powerful simulations. It stands out in the industry as one of the few simulators capable of adjusting power losses according to the junction temperature at the moment of the simulation, providing a more accurate representation of real-world usage. Some competitors traditionally use a fixed value for the junction temperature, leading to over- or underestimated losses. Thanks to our dynamic junction temperature, users get more accurate results.
STPOWER Studio can simulate up to hundreds of seconds per step with Steady State off, which gives engineers enough time to see how their power stage would ramp up and stabilize. They can also run simulations with or without heat sinks, which will help them anticipate form factor and heat dissipation requirements. Users simply select the ST family of devices (ACEPACK or SLLIMM) and the component they will use in their design. Under Setting, designers can tweak the gate resistor values and some thermal properties. Finally, under I/O, users can adjust their mission profile by defining various steps with values such as the output power or the current level, among other things.
A design assistantLet’s take the example of an engineer designing a large motor driver for industrial applications, an inverter for a photovoltaic converter, or an HVAC system. In our example, the motor would use a DC Link voltage of 650 V and an RMS Phase Current of 10 A. For a quick simulation, users can use Steady State ON to analyze performances after reaching a thermal steady state. Then, by choosing Steady State OFF, users can set the duration of the simulation step for a more detailed analysis. Obviously, it will require more computing power on the server and take longer to generate. However, ST reduced rendering times by a factor of 10 over the last releases of STPOWER Studio.
What’s new in STPOWER Studio 4.0? 1-phase full bridge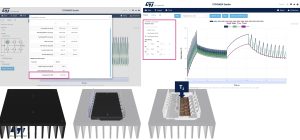
The new version of STPOWER Studio features three new topologies. The 1-phase full bridge will fit single-phase photovoltaic converters or uninterruptible power supplies. As more residential homes and buildings increasingly rely on renewable energy, the ability to store solar energy in batteries is increasingly in demand. Hence, we wanted to ensure that engineers could more rapidly test their designs and reduce their time to market. Similarly, engineers working on an uninterruptible power supply can very quickly anticipate what their design will look like if they adopt an STPOWER device.
1 phase half bridgeSince STPOWER Studio supports a one-phase full bridge topology, it made sense to offer a one-phase half-bridge. This structure is common in DC-AC conversion for smaller solar inverters or motor drivers. Engineers also combine single-phase half-bridge topologies when designing a one-phase to three-phase converter. In fact, while the current version of the simulator focuses solely on DC-AC systems, we are evaluating the addition of DC-DC applications and will update this blog post with more information as they become available.
3-phase 3-level T-NPC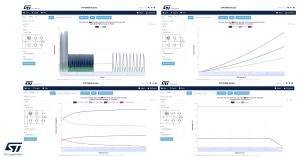
Finally, the 3-phase 3-level T-NPC (T-type Neutral Point Clamped) is increasingly popular because it improves overall efficiency by reducing switching losses thanks to a mechanism that clamps the input voltage at its halfway point. Consequently, only half of the input voltage is applied to each switch, which reduces switching losses. This creative approach greatly benefits high-power systems, such as photovoltaic inverters, power factor inverters, or motor drivers, while ensuring the overall design remains relatively small.
The post STPOWER Studio: 3 new topologies for accurate electro-thermal simulation on STPOWER devices appeared first on ELE Times.
Робоча зустріч з керівництвом Соломʼянського управління поліції
Керівництво поліції Солом’янського району та Київського політехнічного інституту ім. Ігоря Сікорського обговорили питання щодо створення безпекового середовища для студентів.
Weekly discussion, complaint, and rant thread
Open to anything, including discussions, complaints, and rants.
Sub rules do not apply, so don't bother reporting incivility, off-topic, or spam.
Reddit-wide rules do apply.
To see the newest posts, sort the comments by "new" (instead of "best" or "top").
[link] [comments]
Безкоштовний доступ до платформи Research4Life
Понад два роки Міністерство освіти і науки України активно співпрацює з командою проєкту Research4Life щодо надання закладам вищої освіти і науковим установам України безплатного доступу до електронних баз даних та колекцій наукової літератури провідних видавництв.
🤿 Відвідування басейну КПІ для студентів та аспірантів
Кожний студент та аспірант денної форми навчання має можливість 4 рази на місяць безкоштовно відвідувати басейн Спорткомплексу.
🏊 Видача посвідчень для безкоштовного відвідування БАСЕЙНУ КПІ для студентів та аспірантів здійснюється за записом.
SiC MOSFETs drive automotive architectures

Navitas has released a portfolio of third-generation automotive-qualified SiC MOSFETs in D2PAL-7L and TOLL surface-mount packages. Leveraging the company’s trench-assisted planar technology, the Gen-3 Fast SiC devices enable high-speed, cool-running operation for EV charging, traction, and DC/DC conversion.
According to Navitas, the Gen-3 Fast MOSFETs achieve up to 25°C lower case temperatures compared to conventional devices, resulting in an operating life that is up to three times longer than alternative SiC products. The new 650-V MOSFETs, with RDS(ON) ratings ranging from 20 mΩ to 55 mΩ, are designed for 400-V EV battery architectures. The 1200-V Gen-3 Fast MOSFETs, offering RDS(ON) values from 18 mΩ to 135 mΩ, are optimized for 800-V systems.
Both the 650-V and 1200-V ranges are AEC-Q101 qualified in the conventional D2PAK-7L (TO-263-7) package. For 400-V EVs, the 650 V-rated TOLL package offers several advantages: a 9% reduction in junction-to-case thermal resistance, a 30% smaller PCB footprint, 50% lower height, and 60% smaller overall size compared to the D2PAK-7L. These improvements enable high power density and fast switching with minimal package inductance of just 2 nH.
The automotive 650-V and 1200-V G3 Fast SiC MOSFETs are now available for purchase. For more information, contact info@navitassemi.com.
Find more datasheets on products like this one at Datasheets.com, searchable by category, part #, description, manufacturer, and more.
The post SiC MOSFETs drive automotive architectures appeared first on EDN.
Wideband amplifier meets sub-6 GHz requirements

A wideband RF power amplifier module from Elite RF covers signals from 20 MHz to 6 GHz, delivering a maximum output power of 20 W. Elite RF can also combine these 20-watt amplifiers to create higher-power systems, such as 100-watt models, enabling signal transmission over greater distances.
This wideband amplifier is particularly effective in counter-drone systems, addressing growing security and privacy concerns related to drones’ increasing presence across industries. The amplifier supports key communication frequencies in the ISM band, including 433 MHz, 915 MHz, 2.45 GHz, and 5.8 GHz.
The amplifier module features a compact and rugged design for seamless integration into existing systems. With this new addition to its product line of RF amplifiers, which spans frequencies up to 40 GHz, Elite RF aims to provide one-stop power amplifier solutions to RF system integrators.
For more information or to request a price quote, contact sales@eliterf.com.
Find more datasheets on products like this one at Datasheets.com, searchable by category, part #, description, manufacturer, and more.
The post Wideband amplifier meets sub-6 GHz requirements appeared first on EDN.
Wireless combo module targets embedded devices

KAGA FEI’s WKI611AA1 Wi-Fi 6 and Bluetooth 5.4 combo module simplifies the development of low-power embedded devices. With a built-in antenna and multiple certifications, it reduces antenna development time and certification costs for applications such as industrial automation, IoT gateways, surveillance cameras, and smart home appliances.
The WKI611AA1 module employs NXP Semiconductor’s IW-611, a highly integrated dual-band 2.4/5-GHz 1×1 Wi-Fi 6 and Bluetooth/Bluetooth Low Energy 5.4 chip. Its Wi-Fi subsystem includes a Wi-Fi MAC, baseband, and direct-conversion radio with an integrated power amplifier, low-noise amplifier, and transmit/receive switch, eliminating the need for an external RF front-end module. The chip’s independent Bluetooth subsystem supports Bluetooth profiles.
Dedicated CPUs and memories for both the Wi-Fi and Bluetooth subsystems enable real-time, independent protocol processing. Interfaces to external host processors include SDIO 3.0 for Wi-Fi and UART for Bluetooth.
The WKI611AA1 module comes in an LGA surface-mount package that is 25.0×15.7×2.1 mm. It operates from a 3.3-V/1.8V power supply over a temperature range of -40°C to +85°C. Certifications for the combo module include Radio Law MIC (Japan), FCC (USA), and ISED (Canada).
The WKI611AA1 wireless combo module will be available for sampling in December 2024, with mass production slated to begin in May 2025.
Find more datasheets on products like this one at Datasheets.com, searchable by category, part #, description, manufacturer, and more.
The post Wireless combo module targets embedded devices appeared first on EDN.
PCIe 4.0 SSD optimizes system performance

Powered by a Gen 4×4 NVMe controller, the NV3 PCIe 4.0 SSD from Kingston Digital delivers sequential read and write speeds of up to 6000 MB/s and 5000 MB/s, respectively. The drive offers storage capacities of 500 GB, 1 TB, 2 TB, and 4 TB in a compact single-sided M.2 22×80×2.3-mm form factor.
The NV3 solid-state drive provides high-speed performance for tasks ranging from editing to gaming. Its low power consumption and heat generation boost overall system efficiency. The drive’s compact form factor makes it well-suited for thin laptops and small PCs, integrating seamlessly via M.2 connectors.
Endurance of the NV3 SSDs ranges from 160 TBW for the 500-GB model to 1280 TBW for the 4-TB model. Operating over a temperature range of 0°C to 70°C, the drives also feature an MTBF of 2,000,000 hours.
NV3 drives include a 1-year subscription to Acronis True Image software for disk cloning, along with Kingston SSD Manager for monitoring drive health, tracking disk usage, updating firmware, and securely erasing data.
SSD models with 500-GB, 1-TB, and 2-TB storage capacities are available now, with the 4-TB model launching in Q4 2024.
Find more datasheets on products like this one at Datasheets.com, searchable by category, part #, description, manufacturer, and more.
The post PCIe 4.0 SSD optimizes system performance appeared first on EDN.
14-bit scopes boost resolution for general use

The InfiniiVision HD series oscilloscopes from Keysight employ a 14-bit ADC, offering four times the vertical resolution of 12-bit scopes. With a noise floor of 50 µV RMS, they also reduce noise by half compared to other general-purpose scopes.
Covering bandwidths between 200 MHz and 1 GHz, the HD3 series enables engineers to detect even the smallest signal anomalies. Its 14-bit resolution, low noise floor, and update rate of 1.3 million waveforms/s ensure fast and precise debugging across all measurements.
The oscilloscopes provide two or four analog channels, along with 16 digital channels. A deep memory of up to 100 million points captures longer time spans at the full sample rate of 3.2 Gsamples/s, enhancing measurement and analysis results. Additionally, the HD3 series introduces Fault Hunter software, which automatically analyzes signal characteristics based on user-definable criteria.
Prices for the InfiniiVision HD3 series start at $8323 for a 2-channel oscilloscope and $9187 for a 4-channel model.
InfiniiVision HD3 product page
Find more datasheets on products like this one at Datasheets.com, searchable by category, part #, description, manufacturer, and more.
The post 14-bit scopes boost resolution for general use appeared first on EDN.
Interconnect underdogs steering chiplet design bandwagon

The chiplets movement is gaining steam, and it’s apparent from how this multi-die silicon premise is dominating the program of the AI Hardware and Edge AI Summit to be held in San Jose, California from 10 to 12 September 2024. The annual summit focuses on deep tech and machine learning ecosystems to explore advancements in artificial intelligence (AI) infrastructure and edge deployments.
At the event, Alphawave Semi’s CTO Tony Chan Carusone will deliver a speech on chiplets and connectivity while showing how AI has emerged as the primary catalyst for the rise of chiplet ecosystems. “The push for custom AI hardware is rapidly evolving, and I will examine how chiplets deliver the flexibility required to create energy-efficient systems-in-package designs that balance cost, power, and performance without starting from scratch,” he said while talking about his presentation at the event.
Figure 1 Chiplets have played a vital role in creating silicon solutions for AI, and that’s extending to 6G communication, data center networking, and high-performance computing (HPC). Source: Alphawave Semi
At the summit, Alphawave Semi will showcase an advanced HBM3 sub-system designed for AI workloads as well as AresCORE, a 3-nm 24-Gbps UCI integrated with TSMC CoWoS advanced packaging. There will also be a live demonstration of die-to-die (D2D) traffic at 24 Gbps per lane.
LG’s chiplet design
Another chiplets-related announcement involves leading consumer electronics manufacturer LG Electronics, which has created a system-in-package (SiP) encompassing chiplets with processors, DDR memory interfaces, AI accelerators, and D2D interconnect. Blue Cheetah Analog Design provided its BlueLynx D2D interconnect subsystem IP for this chiplet-based design.
Figure 2 Chiplet designs demand versatile interconnect solutions that minimize die-to-die latency and support a variety of packaging requirements. Source: Blue Cheetah
BlueLynx D2D interconnect provides customizable physical (PHY) and link layer chiplet interfaces and supports both Universal Chiplet Interconnect Express (UCIe) and Bunch of Wires (BoW) standards. Moreover, the PHY IP solutions can be integrated with on-die buses using popular standards such as AMBA, CHI, AXI, and ACE.
The D2D interconnect IP is available for 16 nm, 12 nm, 7 nm, 6 nm, 5 nm, and 4 nm process nodes and works on multiple fabs. It also facilitates both standard and advanced packaging while supporting multiple bump pitches, metal stacks, and orientations.
Related Content
- Startup Tackles Chiplet Design Complexity
- How the Worlds of Chiplets and Packaging Intertwine
- Chiplets advancing one design breakthrough at a time
- Chiplets diary: Three anecdotes recount design progress
- Chiplets diary: Controller IP complies with UCIe 1.1 standard
The post Interconnect underdogs steering chiplet design bandwagon appeared first on EDN.
Roborock uses hybrid Time-of-Flight system by Infineon and pmdtechnologies for sweeping and mopping robots
Roborock, a global leader in intelligent home robotics, has launched the intelligent sweeping and mopping all-in-one robot Roborock Qrevo Slim at IFA 2024 in Berlin, equipped with an innovative 3D camera module for navigation and obstacle avoidance using the REAL3 Time-of-Flight (ToF) imager by Infineon Technologies. The technology allows to reduce size and increase reliability. Compared to the body height of traditional sweeping and mopping robots of around 100 mm, the overall height of the Roborock Qrevo Slim is only 82 mm, allowing it to pass through lower and narrower spaces while providing high reliability.
“The integration of our REAL3 ToF into Roborock’s cleaning robot is a great example of how Infineon technology is used to accelerate product innovation in the smart home sector,” said Andreas Kopetz, Vice President Ambient Sensing at Infineon. “Our hybrid ToF solution targets a rapidly growing consumer robotic market, enabling customers to develop unique robot designs while reducing system costs and complexity.”
Roborock has teamed up with Infineon, pmdtechnologies and OMS to develop a next generation robot solution that uses hybrid Time-of-Flight (hToF) to replace the traditional laser distance scanner (LDS) module and obstacle avoidance module. hToF is a combination of Infineon’s REAL3 ToF imager, a dual infra-red illumination sources and pmd’s processing technology to provide a powerful solution for consumer-grade robots. It supports simultaneous localization and map building (SLAM), obstacle avoidance and cliff detection functions in one camera module manufactured by OMS.
“At pmd, we are proud to have played a leading role in the development and optimization of the hybrid Time-of-Flight (hToF) system that powers Roborock’s latest innovation. By contributing our advanced 3D pixels, our expertise in infra-red illumination, and our processing technology into the hToF module, we’ve been able to create a highly efficient, compact solution that significantly enhances navigation and obstacle avoidance capabilities,” said Jochen Penne, Managing Director at pmdtechnologies ag.
The high-resolution depth and vision data is perfectly suited for robust obstacle avoidance algorithms while the open-source SLAM algorithm based on hToF depth data generates high-precision maps, ensuring accurate and reliable navigation. In addition, hToF is working in darkness and strong sunlight. It is computationally efficient and streamlined, requiring e.g. only one Cortex A55 processor core for depth processing and SLAM computation tasks out of eight available cores.
Roborock has pioneered the mass production of LDS technology since 2016 and is promoting it as the standard configuration for sweeping robots. The company is the first to use the hybrid Time-of-Flight system in their new generation of sweeping robots.
The post Roborock uses hybrid Time-of-Flight system by Infineon and pmdtechnologies for sweeping and mopping robots appeared first on ELE Times.
The Demand and Challenges of Electronic Components in the EV Era
As the global demand for sustainable transportation solutions grows, the electric vehicle (EV) market is undergoing unprecedented expansion. Particularly in India, a government-led EV revolution is rapidly transforming this vast market, with electronic components playing a critical role in this change, providing essential technology support for the new generation of electric vehicles.
Current Status and Prospects of the EV MarketThe global electric vehicle market is at a crucial turning point, driven by government policies and technological advancements, with adoption rates rising swiftly. Policies in Europe, China, and the USA, along with increased consumer awareness, have continuously matured these regions’ markets. The International Energy Agency (IEA) predicts that global electric vehicle sales will grow exponentially in the next decade, with market shares expected to rise from 15% in 2023 to 40% in 2030 and surpass 50% by 2035.
In India, the government’s “FAME India” (Faster Adoption and Manufacturing of Hybrid and Electric Vehicles) scheme has set clear targets to accelerate the adoption and production of hybrid and electric vehicles through fiscal incentives and manufacturing support. In 2023, India’s EV sales hit a historical high, particularly in the cost-effective and versatile two-wheeler and three-wheeler segments.
The rapid market growth in India is being propelled by advancements in EV technology, such as reduced battery costs and increased range, as well as by the expansion of charging infrastructure. The government plans to extend charging facilities to more cities and public areas by 2025 to meet growing demand.
时代的电子组件需求和挑战-2.jpg)
- Power electronics systems: including inverters, DC-DC converters, and motor controllers, are the heart of electric vehicles.
- Battery Management Systems (BMS): Given India’s significant temperature variations, BMS must adapt to extreme climates to ensure battery safety and efficiency.
- On-board infotainment and autonomous driving components: As India’s middle class demands more high-tech vehicles, advanced driver-assistance systems (ADAS) and on-board infotainment systems have become standard in new models.
- High voltage and temperature resistance: India’s hot climate demands that electronic components can operate under more extreme conditions.
- Reliability and longevity: In India’s variable roads and climatic conditions, the durability and reliability of components are incredibly crucial.
- Miniaturization and integration: As technology advances, particularly in space-constrained two-wheelers and three-wheelers, the demand for miniaturization and integration grows.
As the world’s leading electronic components distributor, WIN SOURCE has integrated over a million components from more than 3,000 manufacturers. The company’s comprehensive supply chain solutions include global sourcing, obsolete management, cost control, shortage management, alternative solutions, and excess inventory management. Whether facing part shortages, obsolescence, or regular demands, WIN SOURCE is equipped to meet the needs of clients from the EV industry to other high-demand sectors.
With modern warehouses established in Singapore, Hong Kong, Shenzhen, and the Philippines, WIN SOURCE has enhanced its logistics efficiency by 30%, speeding up its response to global markets. The company has also established offices in key global markets, including the USA, Canada, Brazil, the UK, Italy, Germany, Singapore, India, the Philippines, Japan, and South Korea, enabling it to rapidly meet customer needs and solidify its market position. Additionally, the recent opening of a new office in Bangalore, India, further strengthens WIN SOURCE’s global service network and optimizes its local market service capabilities, ensuring that global orders are processed quickly and accurately.
WIN SOURCE will participate in Electronica India 2024, held from September 11 to 13 at the India Expo Centre in Greater Noida, at booth H9.C75. At this event, the company will showcase its extensive product line and innovative solutions, applicable to a wide range of industries beyond just electric vehicles. WIN SOURCE warmly invites all industry colleagues, partners, and experts to visit their booth to discuss collaboration opportunities and exchange the latest insights on market dynamics and technological advancements. This exhibition will demonstrate WIN SOURCE’s ongoing innovation and adaptability in meeting market challenges and seizing industry opportunities, ensuring that clients receive the best quality product selections and services. Additionally, the company’s expert technical team will be available on-site to provide customized consultation services to assist clients with product selection and supply chain management challenges.
ConclusionAs technology continues to advance and the market expands, the development of electronic components will continue to play a pivotal role in supporting the EV revolution. Particularly in India, the market’s unique demands have spurred numerous innovative technologies, which are expected to be promoted and applied globally. The rapid development of India’s EV market presents unprecedented opportunities and challenges for the electronic components industry. Through continuous innovation and collaboration, the electronic components industry can effectively address these challenges and drive the future development of EV technology in India and globally.
Reprinted from WIN SOURCE ELECTRONIC-NEWS
© 2024 Win Source Electronics. All rights reserved. This content is protected by copyright and may not be reproduced, distributed, transmitted, cached or otherwise used, except with the prior written permission of Win Source Electronics.
The post The Demand and Challenges of Electronic Components in the EV Era appeared first on ELE Times.
resonant flyback high voltage generator (not a zvs driver)
![]() | I recently made a high voltage generator that can either output around 20kv at 5mA if I use the resonant capacitor, or around 70kv at 0.4mA if I don’t use the resonant capacitor. The higher current mode, with the capacitor (image 1) creates a hot arc, whereas the lower current mode, without the capacitor, (image 2) can create much higher output voltages. I give the circuit 24V, constant current limited to 7.5A (the constant current part is very important, without the capacitor, it has to run at constant current 7.5 amps) It uses a center tapped coil (5+5) turns on the core of the flyback and 2 MOSFETS (IRFP250N’s). The power side of the circuit (image 3) is very similar to the ZVS driver, although the rest is completely different. This uses a 555 timer to produce a square wave signal, which goes into 2 mosfet cascode drive circuits to drive the MOSFETS. The first cascade drive is fed directly by the signal coming out of the 555 timer, but the 2nd cascade drive is fed with an inverted version of the 555 output (using a BJT). That way, the second mosfet is completely inverted with the first. Using a resonant capacitor will make it extremely efficient, and give out relatively high currents, making a hot arc (image 1). This also makes it operate at ZVS, which makes its waveform practically pretty similar to the ZVS driver, although the huge difference is that this one is not self tuning/resonating, so it doesn’t rely on the resonant capacitor. Removing the resonant capacitor replaces the nice sine wave with inductive spikes. These inductive spikes, even though they only last for less than 1 microsecond, are around 1500V volts, so they can induce a super high voltage (but low current) on the output of the CRT flyback. The actual build is shown in images 4 and 5. [link] [comments] |
Researchers seek to revolutionize neurological disease treatment through intelligent biocomputing
Викладачка КПІ отримала відзнаку від Міжнародного інституту зварювання
Доцентка кафедри зварювального виробництва, кандидатка технічних наук НН ІМЗ КПІ Євгенія Чвертко стала першою жінкою в Україні та у Східній Європі, яка нагороджена відзнакою Міжнародного інституту зварювання — організації, що формує світові тренди в галузі науки та технологій зварювання.
Правила поведінки в укритті
Заходити в укриття потрібно організовано, без метушні. Розміщує людей у відсіках особовий склад ланки обслуговування сховищ та укриттів. Особи, що укриваються, можуть принести в укриття продукти в поліетиленовій упаковці та мати при собі "тривожну валізу".
Fuse failures

Fuses placed in series with the path of some current flow are protective of excessive current flow taking place in that current path. Although fuses are rated for clearing in terms of “I²t” where “I” is in amperes and “t” is time, my personal view is that such ratings are of dubious value from a protective calculation standpoint. To me, a fuse is either a “fast blow” device or a “slow blow” device at whatever amperage applies and which type of fuse to select isn’t always cut and dried, straight forward, or unambiguously obvious.
Some fuses contain their innards inside of glass which allows you to see the current carrying element and some do not. Where glass lets you see that element, actual fuse blowouts can be instructive in terms of the overload condition that led to that blowout and can perhaps lead you to reselecting that fuse’s rating or to fixing some other problem.
Figure 1 An intact fuse and two blown fuses: one from a moderate current overload and one from a massive current overload.
The middle case in the above figure is a fuse that is probably underrated for whatever application it has been serving. Using a somewhat higher I²t device might be a good idea.
However, the lower case shows a fuse that got hit with a blast of overload current that was way, way, way beyond reason and something elsewhere in the system in which this fuse played a role had just plain better be corrected.
John Dunn is an electronics consultant, and a graduate of The Polytechnic Institute of Brooklyn (BSEE) and of New York University (MSEE).
Related Content
- What caused this outlet strip’s catastrophic failure?
- Simple blown-fuse indicator sounds an alarm
- Blown fuse has a meltdown
- Metal eFuse teardown
- PWM circuit uses fuse to sense current
- Managing inrush and system protection for electrical systems
- A new level of circuit protection: The e-fuse
The post Fuse failures appeared first on EDN.
Pages
