Українською
  In English
Microelectronics world news
Oscilloscope probing your satellite

When designing space electronics, particularly during the early prototyping stage or if qualification or flight hardware doesn’t function as intended, the humble oscilloscope is often used to verify the presence, timing and quality of key signals.
When debugging your space electronics using an oscilloscope, many different measurement types are now possible, e.g., voltages, currents, power rails, digital logic, EMC, optical, TDR, and high voltage. For each of these applications, the specification of the probe that makes contact with your device under test (DUT) determines the quality of your test, e.g., its frequency response and bandwidth, how it loads the DUT, whether it matches the input impedance of your scope, where and how you attach the signal and ground contact tips. Often the probe is overlooked or taken for granted. During many of my visits to customers, I have seen the wrong diagnosis due to poor probing techniques or incorrect decision-making because of the specification of the oscilloscope and/or probe. Ultimately, this has impacted the ability of clients to deliver hardware and sub-systems to cost and schedule.
The impact of scope probes on your testAn ideal probe would not load the DUT, would transmit the signal under investigation from its tip to the oscilloscope with perfect fidelity, have zero attenuation, zero capacitance, zero inductance, infinite bandwidth, and linear-phase characteristics at all frequencies.
In reality, a probe is a circuit with its own electrical characteristics and when it makes contact with your DUT, it suddenly becomes part of a larger system with its specification combining with that of the circuit of interest. To make a measurement, the probe must “steal” some energy from the DUT and transfer this to the scope’s inputs such that it does not load the DUT while preventing degradation of the signal to be measured and will not impair the functionality of the DUT. Probes and oscilloscopes have an inherent capacitance creating a low-pass filter that impacts higher frequencies, i.e., bandwidth, slowing rise times. Probes have an intrinsic resistance, forming a voltage divider which reduces signal amplitude. Leads attached to probes add unwanted inductance resulting in overshoot and ringing on the display. Leads can also act as antennae, picking-up electrical noise from the surrounding environment. None of these effects may actually be present in the signal you are trying to measure, so it’s not a surprise if tests are misleading and the diagnosis wrong!
Probing preferencesFor general-purpose testing, the key is to use probes which have a high input resistance to minimize the current taken from the DUT, typically 1 to 10 MΩ, as well as low input capacitance to ensure high impedance at higher frequencies, usually 10 to 30 pF. A low impedance would adversely load the DUT impacting the measured signal level.
As frequencies rise, to avoid reflections due to capacitive and inductive reactances, the source, load, and probe characteristic impedance should be matched, usually 50 Ω. As a guide, an interconnect can be considered a transmission line potentially susceptible to reflections if its time delay, or its critical length, is greater than one third the signal rise time.
Figure 1 compares the signal integrity measured from a 10-MHz clock using both 1 MΩ and 50 Ω input impedances. The former (light blue trace) contains reflections while the fidelity of the latter (green trace) is superior!
Figure 1 The difference in signal integrity due to oscilloscope input impedance. Source: Rajan Bedi
Although I still have several, it’s rare for me to use the much-abused, 50-Ω, BNC oscilloscope coax cable with crocodile clips to accurately test and measure the latest space electronics. However, occasionally I do: the question is, when can you use this $10 “probe” rather than the expensive $10k ones?
A general-purpose solutionLast week I had an FPGA that wasn’t communicating with its JTAG programmer and needed to check the board was receiving the TCK, TMS, and TDI inputs, and outputting TDO. All the expensive, sexy probes were being used, however, due to the low frequency of the JTAG signals, I knew the trusted 50-Ω BNC coax cable (Figure 2) could verify these signals with good integrity.
Figure 2 The ubiquitous 50-Ω BNC oscilloscope coax cable found in most labs. Source: RS PRO
High frequency solutions Knowing the maximum signal frequencyToday’s satellite and spacecraft electronics operate at higher frequencies, with digital signals having faster edges and lower voltages, close to larger switching currents and sensitive analog signals. Many small satellites squeeze all these functions onto one tiny PCB. To accurately measure signals, observe events, and make the correct decisions, the specifications of the probe and the oscilloscope become paramount; in particular bandwidth.
From Fourier analysis, the bandwidth of a digital signal with a 50% duty cycle and a 10 to 90% rise time can be approximated by:
You might not know the edge rates of the digital signals you may have to measure in the future, but if you have an appreciation of the highest frequency of interest, e.g., a clock, an estimate of rise time and hence bandwidth can be calculated. For example, if one assumes rise time comprises 7% of the total period, the signal bandwidth can be estimated as, 5*Fclk, or up to the fifth harmonic!
Knowing the maximum signal frequency allows you select the appropriate oscilloscope and probe bandwidths for accurate measurements. To minimize the in-band effects of their respective 3-dB amplitude roll-offs, these should be 3 to 5 times higher than the largest harmonic contained within the signal of interest.
As an example, for a sine wave with a fundamental frequency of 700 MHz, the oscilloscope and probe bandwidths should each be between 2.1 and 3.5 GHz. Likewise for other analog signals such as modulated carriers, choose bandwidths at least three times larger than its highest frequency component.
For a digital signal with an edge rate of 0.5 ns, its resulting bandwidth can be approximated by 0.35/0.5 = 700 MHz. The measurement bandwidth of the scope and probe should be between 2.1 and 3.5 GHz to accurately capture the fidelity of the fifth harmonic. Similarly, for rise and fall times, if you want to accurately see these transitions, the edge rates of your probe and oscilloscope should be three to five times faster the signal of interest. If you want to validate the specifications of your instrumentation, input a pulse that has rise/fall times three to five times faster!
Impact of scope bandwidthFigure 3 and Figure 4 illustrate the impact of oscilloscope bandwidth on measurement fidelity when verifying a 100 MHz clock with 500 ps edges: a 100 MHz scope only passes through the fundamental frequency while a 500 MHz one captures up to the fifth harmonic preserving the intended waveform, but its own rise time specification is limiting the measurement of the actual signal edge rate. A 1 GHz scope has 20% accuracy while a 2 GHz one offers 3%.
Figure 3 The impact of scope bandwidth on waveform fidelity and rise-time. Source: Keysight
A word of caution, there is such a thing as too much bandwidth as measurements can pick-up high-frequency noise as shown below, impacting the system’s effective number of bits (ENOB). The 20 MHz waveform on the left was captured using a bandwidth of 6 GHz, while the one on the right had 100 MHz. Use the lowest bandwidth possible while still having enough to accurately capture the frequencies contained within your signal of interest. If possible, limit measurement bandwidth using the oscilloscope’s built-in hardware or software filters, and/or the specification of the probe.
Figure 4 The impact of too much measurement bandwidth on waveform noise. Source: Keysight
Debugging a real problemGoing back to my problem of debugging the uncommunicative FPGA using a one meter, 50Ω BNC coax cable as a probe; how did I know this would be fine for verifying the JTAG signals? The delay the signal experiences as it travels down the one metre cable is approximately 5 ns. For rise times longer than 3 * 5 ns = 15 ns (critical length), the resulting bandwidth can be approximated by 0.35/15 ns = 23 MHz. The fundamental frequency of the JTAG signals is around 1 MHz (well below 23 MHz) with a sufficient number of odd harmonics (bandwidth) captured to display the waveforms with good integrity and sharp edges. I also knew the BNC cable has a bandwidth of at least 1 GHz and the oscilloscope 8 GHz. Don’t trash those $10 cables just yet!
Passive probesMany different types of probes can be used with modern digital oscilloscopes enabling a variety of measurement types. Firstly, should you choose a passive or an active probe? The former are often shipped with oscilloscopes, are lower cost, rugged, and good for general-purpose testing often up to several hundred MHz. Internally, these only contain passive components that respond to the signal being measured.
Passive probes have an attenuation factor that impacts DUT loading and the measurement bandwidth: a 1x or 1:1 probe does not change the input amplitude offering better sensitivity for low-voltage signals, whereas a 10x reduces the input magnitude by a factor of ten (Figure 5). These are used to protect the oscilloscope’s maximum rated voltage and offer better SNR as any noise picked up by the probe is also attenuated, thus improving signal quality. The use of a 10:1 probe results in a higher internal resistance, typically 10 MΩ compared to 1 MΩ, which reduces circuit loading. The addition of capacitance in the tip cancels the scope’s input capacitance, increasing bandwidth and improving the measurement of higher frequencies and faster edges.
Check whether your oscilloscope auto-senses the probe attenuation or whether you have to manually switch between these. Passive probes also come with compensation to match the probe and oscilloscope input impedances. Without adjustment, the capacitive loading of the probe may filter out high-frequency components and distort the signal under investigation.
Figure 5 The typical schematic of a 10x passive probe. Source: Rohde and Schwarz
At frequencies beyond 500 MHz, the output capacitance of most passive probes degrades the higher harmonics and edge rates. Furthermore, they can severely load the DUT as the oscilloscope’s input impedance is not significantly higher than the circuit’s output impedance.
Active probes Single-endedActive probes do not use signal power from the DUT, but alternatively utilize wideband amplifiers to enable high-frequency measurements. Active probes have high input resistance and low capacitance, typically less than 1 pF, offering bandwidths up to 20 GHz. The probe in Figure 6 is a single-ended probe, measuring a signal with respect to ground.
Figure 6 The typical schematic of an active probe. Source: Rohde and Schwarz
DifferentialDifferential probes will measure the potential difference between any two points and are suitable for verifying low-amplitude, high-frequency I/O such as LVDS as used by many Earth-Observation imagers. Figure 7 shows a typical schematic for a differential probe and Figure 8 shows an off the shelf active differential probe from Rohde and Schwarz (R&S). Differential probes offer high common-mode rejection over a broad range of frequencies and use an internal differential amplifier to convert the difference between two inputs into a voltage that can be sent to a typical single-ended scope input.
Figure 7 A typical schematic of a differential probe. Source: Rohde and Schwarz
Figure 8 An active differential probe. Source: Rohde and Schwarz
Power-rail probePower-rail probes allow you to measure AC ripple, high-frequency noise, and transients at high bandwidths on supply voltages with large DC offsets, and then analyze the spectrum of this interference. EMC probes enable electric and magnetic (E&H) near-field debugging of EMI issues, while current probes provide a non-invasive method for measuring current flow through a conductor. A DC probe uses a hall-effect sensor to detect the magnetic field generated by a current as it passes through the probe’s ferrite core, while an AC probe uses a transformer to measure current flow through its core.
Figure 9 shows a recent current measurement from an Earth-Observation payload to verify its in-rush behavior at power-up. Thank you to my friends Giovanni and Nick from R&S UK for helping me with this test.
Figure 9 Oscilloscope current probe measuring payload in-rush current at power-up. Source: Rajan Bedi
One issue often overlooked is the parasitic effect of the tips probing the DUT, known as the “connection bandwidth”. How and where you probe is equally as important as the specification of your test equipment: long connections degrade the measurement bandwidth, slowing edges, as well as adding unwanted inductance, resulting in ringing and distortion when measuring high-frequency signals. These may not actually exist in the circuit under investigation! Parasitic components to the left of the point labelled VAtn below determine the quality of the actual measurement. Figure 10 is a useful image from Keysight highlighting the impact of probe-tip length on measurement bandwidth.
Figure 10 The impact of probe-tip length on measurement bandwidth. Source: Keysight
Top tipsHere’s my Top Tips when considering oscilloscope probes to test your space electronics:
- Before choosing a probe, understand the statistics of the signals to be measured—amplitude, frequency, bandwidth, and edge rates—and then specify the probe as described.
- Ensure the probe is compatible with your scope’s input impedance.
- Ensure the probe does not adversely load the DUT and compensate passive probes.
- For single-ended probing, do not confuse the signal and ground measurement points—I did this once and killed an FPGA!
- Ensure the probe has a better or comparable bandwidth to the oscilloscope.
- Use short leads/tips to maximize probe bandwidth and minimize parasitic components.
- Specify the required measurement bandwidth but avoid too much to minimize noise.
- Check common-mode rejection before testing.
- Consider its ergonomics/physical design, order a holding fixture if you run out of hands!
- Keep a few traditional BNC cables in the lab. in case your colleagues won’t share.
To conclude, the humble oscilloscope is often used to verify the presence, timing and integrity of key signals during the early prototyping stage or, if qualification or flight hardware doesn’t function as intended. Many different tests are now possible using your scope and choosing the correct probe is critical. The choice will require an understanding on how its specification reconciles and interacts with your DUT, its parasitic effects, and how and where the probe is used, as this will all impact the quality of your measurements.
We could probe further , but I’m off to the lab: until next month, the person who shares their best oscilloscope probing story will win a Spacechips’ Training World Tour tee-shirt.
Spacechips will be teaching its training course, Testing Satellite Payloads, next year and you can contact, events@spacechipsllc.com, for more information.
Dr. Rajan Bedi is the CEO and founder of Spacechips, which designs and builds a range of advanced, AI-enabled, L to K-band, ultra-high-throughput transponders, Edge-based on-board processors, SDRs and MMUs for telecommunication, Earth-Observation, navigation, internet, space-domain awareness, space-debris retrieval and M2M/IoT satellites. The company also offers Space-Electronics Design-Consultancy, Technical-Marketing, Business-Intelligence, Avionics Testing and Training Services. You can also connect with Rajan on LinkedIn.
Spacechips’ Design-Consultancy Services develop bespoke satellite and spacecraft hardware and software solutions, as well as advising customers how to use and select the right components, how to design, test, assemble and manufacture space electronics.
Related Content
- Spacecraft on-board computing using rad-hard ARM MCUs
- Testing times for design engineers
- How to choose passives for space-grade switching regulators
- Pesky parasitics
- Understanding and applying oscilloscope measurements
- Digital vs. analog triggering in oscilloscope: What’s the difference?
- Measuring pulsed RF signals with an oscilloscope
The post Oscilloscope probing your satellite appeared first on EDN.
ROHM and TSMC collaborating on development and volume production of GaN power devices for EVs
onsemi acquiring Qorvo’s United Silicon Carbide subsidiary and SiC JFET technology for $115m
POET appoints Bob Tirva as board director and Audit Committee member
EPC launches GaN FET-based reference design for solar PV optimizers
PCBWay 7th Project Design Contest: Unleash Your Creativity in Electronics and Engineering
PCBWay, a leading platform renowned for its high-quality PCB manufacturing and prototyping services, continues to foster innovation and collaboration in the tech community through its annual Project Design Contest. With six successful editions behind it, the PCBWay Design Contest has become a hub for enthusiasts, professionals, and makers to showcase groundbreaking ideas in electronics, mechanical engineering, and embedded systems. PCBWay’s commitment to open-source innovation and community engagement makes it the go-to platform for creators worldwide.
For the 7th PCBWay Project Design Contest, the platform takes a step further by introducing the STM32 theme, alongside the well-established categories of Electronics and Mechanical Design. This year’s competition offers exciting opportunities for participants to demonstrate their skills, gain recognition, and win fantastic prizes.
Contest Details and Categories
1. Electronic Project
Electronic project invites submissions ranging from simple circuits to complex IoT and embedded systems. Whether your project involves analog and digital electronics, power systems, or audio solutions, PCBWay provides a platform to share your creativity.
2. Mechanical Project
Mechanical engineers and designers can participate by submitting projects such as robotics casings, drone structures, or any innovative mechanical design. The focus is on functionality, aesthetics, and innovation.
3. STM32 Project
The newest addition to the contest, STM32 Project category celebrates projects utilizing STM32 series chips. These versatile microcontrollers power everything from smart home devices to robotics. Participants in this category stand a chance to win additional exclusive rewards.
Key Dates and Timeline
Submission Period: September 2, 2024 – January 19, 2025
Review Period: January 20, 2025 – February 28, 2025
Results Announcement: March 10, 2025
Prizes to Fuel Innovation
PCBWay recognizes outstanding contributions with generous rewards, including cash, coupons, and cutting-edge tech gadgets:
Main Prizes
- First Prize:
$1500 Cash + $200 Coupon + Palm-sized Robot Dog
- Second Prize (2 Winners):
$1000 Cash + $100 Coupon + Raspberry Pi 5 (4GB)
- Third Prize (3 Winners):
$500 Cash + $50 Coupon + OpenMV4 H7 R2 Smart Camera
Special Prizes
Popular Design Prize (10 Winners): $100 Cash + $20 Coupon + Multimeter ZTY890DPro
Participation Prize:
Raspberry Pi Pico
STM32 Great Project Prize
Exceptional projects incorporating STM32 chips will receive a Six-Claw Multifunctional Aluminum Alloy Soldering Table.
How to Participate
Register and Submit:
Visit the PCBWay competition page, create a project, and upload your designs under the appropriate category: Electronic, Mechanical, or STM32.
Provide Original Design Files:
Include comprehensive documentation such as Gerber files, schematics, code, and mechanical designs.
Promote Your Project:
Share your submission to attract community support, as project popularity plays a role in evaluations.
Evaluation Criteria
Submissions are judged on four key aspects:
Project Design:
Complexity and innovation are paramount.
Project Description:
Detailed explanations, videos, and open-source materials enhance understanding.
Popularity:
Community engagement adds value.
Social Significance:
Projects with a positive societal impact are highly encouraged.
Rules and Regulations
1. Originality
All entries must be original and uploaded within the specified timeframe. Late submissions will be disqualified.
2. Intellectual Property
Participants retain the intellectual property rights to their projects and must ensure their submissions do not infringe on third-party intellectual property.
3. Required Files
Each submission must include original design files:
- Electronic Projects: PCB designs, Gerber files, BOM, schematics, layouts, and code.
- Mechanical Projects: Mechanical design files, CNC machining files, and other relevant documentation.
- Participants are encouraged to share comprehensive files to help judges understand their creativity better.
4. Submission Process
Click the “Participate Now” button on the PCBWay competition page.
Alternatively, create a project in the PCBWay community and submit it under the appropriate category: Electronic, Mechanical, or STM32.
5. Participation Rewards
Once an entry is approved, 500 points will be credited to the participant’s PCBWay account, which can be redeemed for a Raspberry Pi Pico in the store.
6. STM32 Additional Rewards
Projects utilizing STM32 chips are eligible for additional rewards based on evaluation by judges. Not all STM32 projects will receive these rewards.
7. Organizer Rights
PCBWay reserves the right to interpret and modify event rules and plans. For any inquiries, participants can contact anson@pcbway.com.
PCBWay: Empowering Makers Worldwide
PCBWay has been at the forefront of advancing technology through accessible prototyping and manufacturing services. Their dedication to quality, innovation, and open-source collaboration makes them a trusted partner for creators. By organizing the 7th Project Design Contest, PCBWay continues to nurture talent and inspire the global electronics and engineering community.
Whether you’re an electronics enthusiast, a mechanical design wizard, or an STM32 expert, this contest is your chance to shine. Join PCBWay in pushing the boundaries of innovation and creativity! For more details, visit PCBWay’s official contest page and let your imagination lead the way.
The post PCBWay 7th Project Design Contest: Unleash Your Creativity in Electronics and Engineering appeared first on Electronics Lovers ~ Technology We Love.
Imec integrates InP chiplet on 300mm RF silicon interposer, yielding 0.1dB insertion loss at 140GHz
Qorvo receives GSA award for Most Respected Public Semiconductor Company
The Blink Mini: AC-powered indoor camera-based security

One portion—battery-operated outdoor-optimized devices—of Blink’s security camera product line (bought by Amazon in December 2017, followed shortly thereafter by Amazon’s acquisition of Ring, and with both brands still viable market options), has already gotten plenty of coverage attention from yours truly. But the product line in its entirety is much more diverse, both generationally (battery-operated outdoor cameras are now in their fourth generation, for example) and operationally (both indoor and outdoor variants, both battery- and AC-powered). The Blink Mini 2, another example, is intended for both indoor and outdoor AC-powered setups:
And here’s the Pan-Tilt variant of the original Blink Mini:
That said, the first-generation Blink Mini, which was introduced in early 2020 and is still sold by Amazon, is what we’ll be tearing down today:
Like its siblings, it comes in both white and black color options:
Key to my acquisition motivation was the following excerpt from an early review:
It comes with free cloud storage through the end of this year, and if you already have a Blink account through an older Blink camera, you’ll continue to get free cloud storage as a perk.
At intro, the Blink mini cost $35 (for one) or $65 (for a two-pack), plus the optional Sync Module 2 for an additional $35. Why’s the Sync Module optional, in this case, versus required for my battery-operated cameras? That’s because, as I explained in detail in one of my initial posts in the series and summarized in the subsequent sync module teardown:
Each of the cameras in a particular network implementation connects not only the common LAN/WAN router over Wi-Fi, but also to a separate and common piece of hardware, the Sync Module, over a proprietary long-range 900 MHz channel that Blink refers to as the LFR (low-frequency radio) beacon.
The Sync Module also connects to the router over Wi-Fi. And the cameras, which claim up-to-2 year operating life out of a set of two AA lithium batteries, are normally in low-power standby mode. If the cameras’ PIR motion sensing is enabled, they’ll alert the Sync Module over LFR when they’re triggered, and the Sync Module will pass along the operating status to “cloud” servers to prepare them to capture the incoming video clip.
Similarly, when you want to view a live stream from a particular camera using the Blink app for Android or iOS, or over an Amazon Echo Show or Echo Spot (or through the Echo Show mode available in recent-edition Amazon Kindle Fire tablets), it’s the Sync Module you’ll be talking to first. The Sync Module will pass along the app’s request and activate the selected camera, turning it back off again afterwards to preserve battery life. The Sync module itself is AC-powered, via a USB power adapter intermediary.
The difference this time, of course, is that the cameras themselves are also AC-powered; low-power standby operation to maximize battery life therefore isn’t relevant in this situation. As such, the key enhancement supported by the 2nd-generation Sync Module, at least for the Blink Mini, is local storage to a plugged-in USB flash drive, for folks who don’t want to spring for a $3/month/camera (!!!) cloud storage subscription and aren’t (like me) “grandfathered” legacy users. Thereby explaining why the Sync Module 2 isn’t bundled with Blink mini cameras, as it is with battery-powered alternatives, but instead is a now-$49.99 accessory (that said, I just bought a “nonfunctional, for parts only” one off eBay for future-teardown purposes!):
In January 2023, I picked up a ”used-good” condition Blink mini 2-camera kit for $18.99 (plus tax) total from Woot, an Amazon subsidiary company. The original purchaser’s shipping sticker was still affixed to the front box panel when I got it (a separate shipping box apparently hadn’t found use); I’ve done my best to peel it off in the first of the photos that follow:
Flip open the box:
and the two cameras inside come into view:
Here’s today’s patient, along with its accoutrements: some optional mounting hardware:
The USB-to-micro-USB power cable:
The 5-W output “wall wart”, as usual accompanied by a 0.75″ (19.1 mm) diameter U.S. penny for size comparison purposes:
And the camera again, now standalone save once more for the aforementioned US penny (the camera’s dimensions are 1.9 x 1.9 x 1.3 in/48 x 48 x 34 mm, and it weighs 1.7 oz/48 grams with an additional 1.5 oz/42 grams for its mount). Front:
Left side:
Back, revealing the micro-USB power input (the QR code finds use during initial setup):
Right side:
Let’s get that protective clear plastic off:
Still reflectively shiny. That said, the LEDs to either side of the lens (green/red to the left, blue to the right) are a bit less obscured now, as is the microphone below the lens. Not visible here but located in the upper right corner of the lens is an 850 nm infrared LED for “night vision” purposes. And the lens has a 110-degree viewing angle and captures 1080p images:
Up top is the speaker:
And down below are rubberized pads to prevent the camera from marring whatever surface it’s sitting on, plus a (comparatively) heavy metal disc to keep it from tipping over:
You probably also noticed the two optional mounting-screw holes in the bottom-view photo, which are related to the earlier-shown baggie-enclosed hardware. Here’s how you gain access to the holes’ topsides:
And under the camera itself is a hardware reset switch:
Time to dive inside. As I go along, particularly when I get to the PCB and lens assembly, you might want to also periodically reference my earlier teardown of the Blink XT battery-operated outdoor camera for compare-and-contrast purposes. Let’s start by extracting that metal disc:
Although, after all that effort, we didn’t get very far:
It turns out, however, that a firm tug is all it takes to separate the base from the camera body:
Giving us now a clear view of, among other things, the camera’s FCC ID (2AF77-H1931660):
If you visit the FCC site using the above link, you’ll see that the applicant name is “Immedia Semiconductor LLC”. As mentioned in previous Blink-themed posts, Immedia was originally founded in 2009 as a chip supplier but pivoted to become a consumer electronics system supplier in 2014, branded as Blink, with a highly successful Kickstarter unveil that July. 3.5 years later, as previously mentioned, Amazon acquired the company, and Immedia Semiconductor, LLC continues to operate as an independent subsidiary (thereby explaining the FCC info).
Onward. Fortunately, prior to striving to get inside myself, I’d done a bit of online research. This intrepid hacker had spectacularly mangled her camera’s case during disassembly:
only afterward, alas, stumbling across a video that greatly simplified (not to mention doing much less damage in) the process:
For likely obvious reasons, I followed in the second person’s footsteps:
Voila:
Here’s the now-exposed inner case topside speaker:
and underside:
The notched grooves on both sides; right:
and left:
And the inside-outer-case clips that originally fit into those grooves. This time, as you can see from the respective damage-or-not, the thinner, more flexible iSesamo “spudger” panned out more intact than its stronger but also thicker and more rigid Jimmy counterpart had. Right:
and left:
Oh well, our objective is now in sight, and any collateral damage done along the way is relative:
I want to first draw your attention to the two large gold-color rectangular PCB contacts in the upper right, to the right of an unpopulated seeming screw hole at the top of the PCB. Note their proximity to the speaker vent holes at the top of the outer case? Now let’s look at the inside of the inner case:
Yep, that’s again the speaker at the top. And when assembled, those two “spring” contacts mate with their PCB counterparts to drive it with an audio signal.
Back to the PCB. Although that top hole doesn’t have a screw in it, the two on the sides are populated. Let’s fix that:
We have liftoff:
Going forward I’ll be referring to this as the “rear” PCB, to differentiate it from the “front” PCB still currently existent in the outer case. Looking first at the “rear” PCB’s now exposed frontside:
there’s, for example, the connector at bottom right that originally mated it to the “front” PCB and held it in place even after the screws were removed, necessitating the earlier-shown Jimmy-as-crowbar to prod it into detaching. In the upper right is a Faraday Cage whose contents we’ll see shortly. In the upper left are Immedia Semiconductor markings. And in the center is the lense assembly; the two-wire harness routing to it suggests that, as in past teardowns of products like this, there’s likely an IR filter normally between the lens and image sensor that can selectively be moved out of the way for “night vision” applications.
Back to the backside of the “rear” PCB:
Note again the two large contacts in the upper right that feed the speaker. Accompanying them are numerous smaller contacts spread across the PCB, which presumably act as test points, assembly-line firmware programming landing pads, and the like. And speaking of which, in the lower left corner is a Winbond W25Q32JW 32 Mbit SPI serial NOR flash memory.
Two more screws remain in the center of the PCB; I’m guessing from past experience that they hold the other-side lens assembly in place in front of the image sensor. Let’s test my hypothesis:
Yep (to the lens-assembly removal scheme, moveable IR filter inside and sensor underneath):
And here’s the as usual dallop of dried glue that holds the lens in position after its focus and other optics characteristics are fine-tuned on the assembly line:
Before switching our attention to the “front” PCB, let’s revisit that Faraday Cage:
Inside is the application processor, Immedia’s AC1002B. Since it’s Amazon-proprietary, there’s unsurprisingly no public documentation available, aside from a brief mention in the camera documentation of “4 cores / 200 MHz”. That said, I’ll note that it’s different than the Immedia ISI-108A SoC I found in my earlier Blink XT teardown , although the Blink XT2 successor switched to it. And while I’m on the teardown-comparison topic, the flash memory is 4x the capacity of that seen previously.
Last, but not least, let’s switch our attention to the remaining “front” PCB:
Two more screws to go (by the way, note that in addition to the earlier mentioned unpopulated screw hole site at the top of the device, there’s now an additional one at the bottom!):
And the “front” PCB is now also free:
It’d be hard to miss the PCB-embedded antenna in the upper right corner, even if you tried to overlook it! The markings on the shiny IC below it are pretty faint, so take my word when I tell you that the first line identifies it as Infineon’s CYW43438 1×1 single-band Wi-Fi 4 (802.11n) + Bluetooth® 5.1 combo chip (unsurprising given its antenna proximity; it seems that the IC’s Bluetooth facilities are unused in this design). Below that, at the lower right edge, is the reset switch. In the lower left is the other end of the PCB-to-PCB connection scheme. And in-between them, directly below the lens “hole”, is the MEMS microphone, whose input port is on the other side of the PCB. Speaking of which…
In the upper right quadrant is the earlier mentioned 850 nm infrared LED for use in dim ambient light settings. To clarify, it’s not a PIR module, as was found in the earlier Blink XT teardown. in this particular camera model case, an alternative technique called Pixel Difference Analysis (PDA) finds use in detecting object motion in the scene. On either side of the lens “hole” are the two LEDs, respectively labeled D2 (blue to right) and D4 (green/red to left). And below the lens “hole” is the MEMS microphone input port. By the way, I’m struck by how many seemingly unpopulated-component solder pads there are on both sides of this PCB. Readers, agree?
With the PCBs out of the way, all that’s left to do is push the front panel out of the case:
Note that there’s an additional “hole” site above the lens opening, which is currently plastic-filled. As with those unpopulated component sites I just mentioned, along with the unused screw holes I noted earlier, whenever I see something like this, I wonder what it was originally intended to serve as…a second microphone for ambient noise-cancellation purposes, perhaps?
I’ll close with a confession. At some point in these final teardown steps, the crooked rubbery white plastic widget in the lower right corner of the previous photo fell off, and I was admittedly baffled as to what function it served…until I retraced my earlier disassembly steps (and photos) and remembered that it was the inherently elastic interface between the PCB-mounted hardware reset switch and the outside world:
And speaking of disassembly steps…since my removal of the Faraday Cage “lid” and more general dissection were so “clean” (mangled side clip aside), after this writeup is published I’m going to strive to tediously and patiently reverse course and successfully reassemble the device back to a fully functional state. Wish me luck; I’ll post the outcome in the comments. And as always, I also look forward to reading your thoughts there; thanks in advance for them!
—Brian Dipert is the Editor-in-Chief of the Edge AI and Vision Alliance, and a Senior Analyst at BDTI and Editor-in-Chief of InsideDSP, the company’s online newsletter.
Related Content
- Blink: Security cameras with a power- and bandwidth-stingy uplink
- Blink Cameras and their batteries: Functional abnormalities and consumer liabilities
- Teardown: Blink XT security camera
- Blink: Security camera system installation and impressions
The post The Blink Mini: AC-powered indoor camera-based security appeared first on EDN.
"Cool" Project I worked on for Energy Harvesting
![]() | https://oshwlab.com/zachjonbutler1/bq25504 Using a peltier to generate power to drive the blue LED off the temperature differential from an ice cube and my room temperature air. The IC used is a boost converter/ battery charge controller that works down as low as 0.25V input after started. Thought it was neat and decided to share incase anybody wants to use it the files are there for free you just need to tweak the values for the resistors that set your voltage outputs and cutoffs. [link] [comments] |
I made an led matrix using
I made it using an atmega8a, 2 74hc595 shift registers, a cd4017 counter. I did it as a hobby.
I also think i broke a 74hc595 but I had an spare. I'm not an electronic engineer so if it's not perfect thats the reason.
[link] [comments]
Jumpstarting a laptop motherboard
![]() | submitted by /u/Faloin [link] [comments] |
Advantest announces KGD test cell for power semiconductors
What’s LVGL, and how it works in embedded designs

Light and Versatile Graphics Library (LVGL) is steadily making inroads in the graphics realm by efficiently facilitating graphical user interface (GUI) designs in small, resource-constrained, and battery-powered devices such as wearables, e-bikes, navigation systems, instrument clusters, medical gadgets, and more.
Graphics IP suppliers are increasingly partnering with LVGL to optimize GPU performance and expand graphic processing capabilities for a wide range of embedded applications. But who’s LVGL? It’s the company behind the free and open-source graphics library for embedded systems; it helps developers create GUIs for microcontroller units (MCUs), microprocessor units (MPUs), and display processors.
Figure 1 LVGL has no external dependencies, which makes its porting incredibly simple.
LVGL, written in C, allows embedded developers to create modern and visually appealing user interfaces in embedded applications. It works with various processors and operating systems and enables developers to keep code size and memory usage to a minimum. It can be used with any RTOS and bare-metal setup and quickly adapts to unique project needs.
What IP suppliers do is integrate their GPU solutions into LVGL’s graphics ecosystem; so, developers can build sleek, responsive interfaces without compromising on performance or power efficiency. The integration of LVGL into GPUs is transforming the embedded UI landscape in resource-constrained devices like MCUs.
Design case studies
Take the case of Think Silicon, a supplier of ultra-low-power GPU IPs for embedded systems and an Applied Materials company. It’s teaming up with LVGL to develop high-performance, low-power graphics libraries for MCUs. As a result, the software development kit for its NEMA GPUs will be able to accelerate LVGL’s graphics library by up to 5x compared to software-only rendering.
Figure 2 Think Silicon has combined LVGL’s lightweight open-source graphics library with its NEMA GPU-Series.
VeriSilicon, a Shanghai, China-based supplier of embedded GPUs, has also partnered with LVGL to facilitate seamless integration of 2D, 2.5D, and 3D content in embedded applications. In conjunction with LVGL’s graphics library, VeriSilicon aims to advance 3D rendering capabilities in the GUI designs.
Actions Technology, a Zhuhai, China-based firm developing chips for AIoT applications, has incorporated VeriSilicon’s GPU into its smartwatch system-on-chip (SoC) design. Tim Zhang, GM of Actions Technology’s Wearable and Sensing Business Unit, acknowledges the importance of LVGL’s graphics technology contribution in delivering rich 3D graphics in its smartwatch SoC.
Figure 3 Actions Technology has incorporated VeriSilicon’s LVGL-enabled GPU in its smartwatch SoC.
Embedded GPUs are now serving a wide range of applications, from wearables and infotainment to micro-mobility and AIoT. Here, the integration of LVGL in GPUs enables users to create visually appealing UIs across a wide variety of hardware platforms.
Related Content
- Re-imagining Imagination Technologies
- GUI Development: Embedding Graphics
- CAST launches graphics acceleration IP cores
- Imagination outstrips all other GPU IP suppliers
- Two-digit nanosecond latency CXL IP addresses GPU memory expansion
The post What’s LVGL, and how it works in embedded designs appeared first on EDN.
Coherent, SkyWater and X-Fab all agree preliminary terms for US CHIPS Act funding
Securing EMI Resilience with Software-Driven Smart Electronic Fuses
By: Giusy Gambino, Sebastiano Grasso, and Filippo Scrimizzi STMicroelectronics
Integrated smart power devices are becoming increasingly crucial in automotive systems due to their ability to combine power management with advanced diagnostic and protection features. When a fault occurs, these features enable the connected control unit to react promptly and effectively, protecting both the vehicle and its occupants. Smart power devices are often responsible for critical tasks within a vehicle and are gradually replacing conventional components such as fuses and relays, and switches.
Electronic fuses, a prominent type of smart power device, are particularly remarkable for their reliability under harsh environmental conditions, including high operating temperatures and mechanical stress. Additionally, they have to withstand various forms of electromagnetic interference (EMI) that can affect their normal functionality. This is especially crucial in safety-critical applications like airbag control modules or Anti-lock Braking Systems (ABS), where precise operation is vital to avoid hazardous conditions and ensure functional safety.
Testing Procedure for EMI
To ensure the correct operation of electronic products, they need to be tested for electromagnetic emissions that may negatively affect nearby equipment. These emissions fall into two categories:
- Conducted noise, which travels through supply or data/control cables.
- Radiated noise, which propagates through free space.
CISPR 25 is the standard developed for vehicles and boats to protect on-board receivers from such emissions. It outlines both limits and methods of measurement for equipment on board.
Conducted emission limits typically cover a frequency range of 150 kHz to 200 MHz, although this range may extend down to 9 kHz. The limits are defined in terms of average, quasi-peak and peak values.
Peak detection retains the peak value of each harmonic in an emitted signal, indicating the worst-case scenario. Average detection provides the average amplitude of each signal component across its period. Quasi-peak detection weighs each component based on its repetition rate: the faster the repetition rate, the higher the weight given to that component.
The output response of the three detectors to two similar pulsed signals is shown in with the top one having a higher repetition rate.
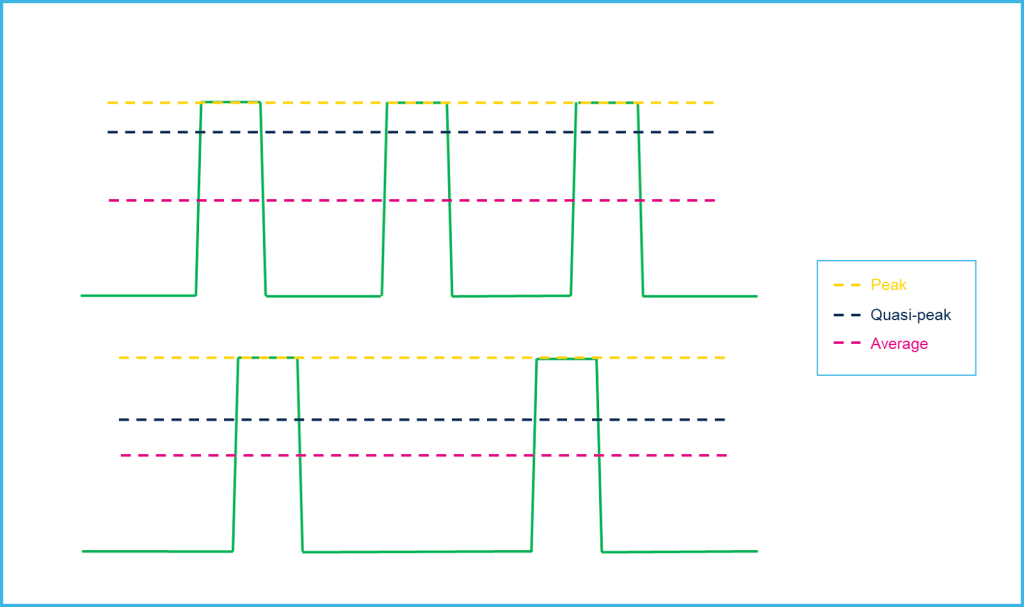
The above picture shows that the quasi-peak detector has a higher voltage output when the event occurs more frequently.
To determine the conformance of the Equipment Under Test (EUT) with the specified CISPR 25 limits, the following guidelines should be followed (Fig. 2).
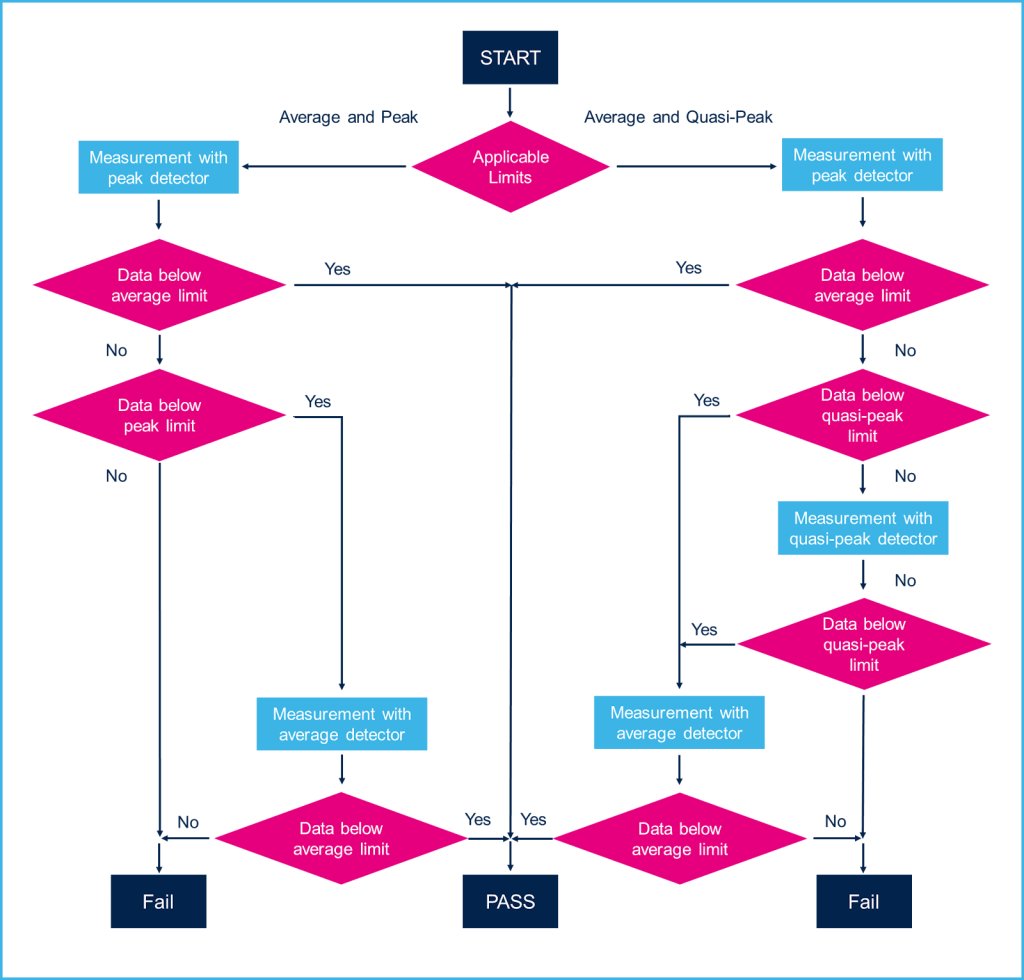
In all cases, the EUT should conform to the average limit. For frequencies where both peak and quasi-peak limits are defined, the EUT should conform to either the peak or the quasi-peak limits, as specified in the test plan. For frequencies where only peak limits are defined, the EUT must conform to the peak limit.
For CISPR 25, emission limits are divided into Class 1, 2, 3, 4, and 5 products, specifying how to measure the noise using peak, quasi-peak, and average detection methods (Tabs. 1 and 2).
Service/Band | Frequency MHz | Levels in dB (µV) | ||||
Class 1 | Class 2 | Class 3 | Class 4 | Class 5 | ||
Peak | Peak | Peak | Peak | Peak | ||
BROADCAST | ||||||
LW | 0.15 – 0.30 | 110 | 100 | 90 | 80 | 70 |
MW | 0.53 – 1.8 | 86 | 78 | 70 | 62 | 54 |
SW | 5.9 – 6.2 | 77 | 71 | 65 | 59 | 53 |
FM | 76 – 108 | 62 | 56 | 50 | 44 | 38 |
TV Band I | 41 – 88 | 58 | 52 | 46 | 40 | 34 |
MOBILE SERVICES | ||||||
CB | 26 – 28 | 68 | 62 | 56 | 50 | 44 |
VHF | 30 – 54 | 68 | 62 | 56 | 50 | 44 |
VHF | 68 – 87 | 62 | 56 | 50 | 44 | 38 |
Service/Band | Frequency MHz | Levels in dB (µV) | ||||
Class 1 | Class 2 | Class 3 | Class 4 | Class 5 | ||
Average | Average | Average | Average | Average | ||
BROADCAST | ||||||
LW | 0.15 – 0.30 | 90 | 80 | 70 | 60 | 50 |
MW | 0.53 – 1.8 | 66 | 58 | 50 | 42 | 34 |
SW | 5.9 – 6.2 | 57 | 51 | 45 | 39 | 33 |
FM | 76 – 108 | 42 | 36 | 30 | 24 | 18 |
TV Band I | 41 – 88 | 48 | 42 | 36 | 30 | 24 |
MOBILE SERVICES | ||||||
CB | 26 – 28 | 48 | 42 | 36 | 30 | 24 |
VHF | 30 – 54 | 48 | 42 | 36 | 30 | 24 |
VHF | 68 – 87 | 42 | 36 | 30 | 24 | 18 |
LW stands for Long Waves
MW for Medium Waves
SW for Short Waves
TV Band I for TeleVision broadcast Band 1
CB for Citizens Band
VHF for Very High Frequencies.
The testing procedure ensures that both peak and average measurements are checked for compliance with Class 5 standards. If any measurements fail, the EUT is evaluated against a lower class.
Conducted Electromagnetic EmissionThe conducted Electro-Magnetic Emission (EME) is the noise current generated by the EUT that propagates through the harness to other components/systems or power grid. This noise current can be measured using either the voltage method or the current method.
The voltage method is carried out with the measurement setup displayed in Fig. 3.
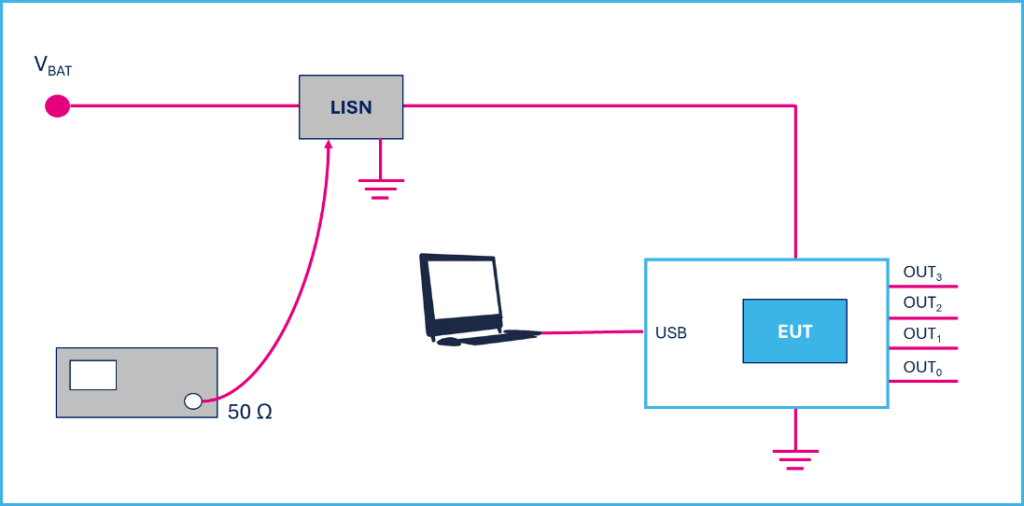
where:
LISN stands for Line Impedance Stabilization Network.
Experimental ResultsThe experimental data have been collected for the STi2Fuse product VNF9Q20F, which is a 4-channel monolithic electronic fuse fully programmable through serial peripheral interface (SPI). This device embeds sophisticated digital protection and diagnostic mechanisms, which include a unique i2t feature for harness protection.
The following testing conditions have been considered:
- VBAT = 13 V
- TAMB = Room temperature
- All channel in Fail Safe mode (off)
- Channel 2 driven by direct input with DC (5 V) and PWM (100 Hz, 50% duty cycle) loaded with 27 W + 5 W bulbs.
When channel 2 is turned on and it is driven by direct input with 5 V DC voltage and a PWM signal with 100 Hz frequency and 50% duty cycle, the noise detector measurements for peak and average methods are shown in Fig. 5 and compared with CISPR 25 limit relevant to class 5.
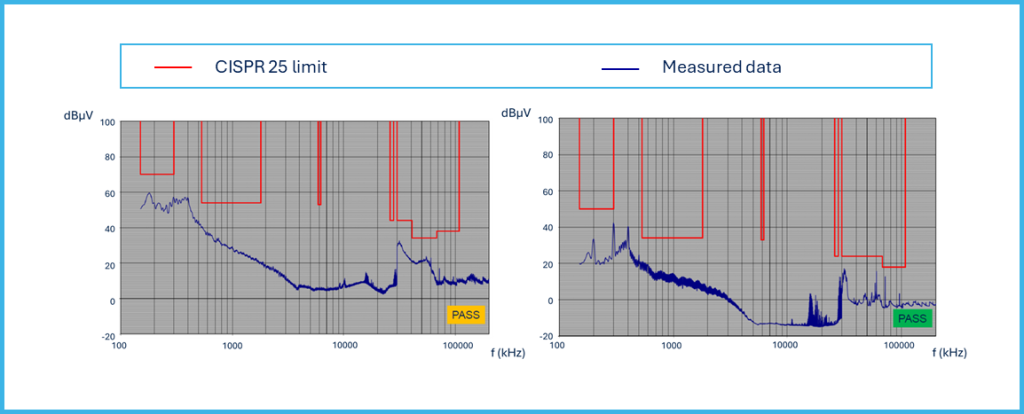
The electronic fuse VNF9Q20F is able to pass all the limits imposed by CISPR 25 for both peak and average limits in class 5.
ConclusionsThe EMI behavior of a smart electronic STi2Fuse device has been investigated, demonstrating compliance with the CISPR 25 standard under class 5 specifications.
The STi2Fuse products are critical components in modern vehicles, providing overcurrent protection and ensuring the safe operation of electrical circuits. Compliance with the CISPR 25 standard, particularly under class 5 specifications, is essential for these devices to ensure they do not interfere with other components and equipment within the vehicle.
References[1] VNF9Q20F, 4 channel high-side driver with STi2Fuse protection for automotive power distribution applications.
[2] CISPR 25:2021 International Standard, Vehicles, boats and internal combustion engines – Radio disturbance characteristics – Limits and methods of measurement for the protection of on-board receivers, Edition 5.0, Dec. 2021.
[3] R. Letor, R. Crisafulli, Smart Power devices and new electronic fuses compliant with new E/E architecture for autonomous driving, AEIT International Conference of Electrical and Electronic Technologies for Automotive (AEIT AUTOMOTIVE), 02-04 July 2019
The post Securing EMI Resilience with Software-Driven Smart Electronic Fuses appeared first on ELE Times.
Pleasant surprise finding a raspberry pi while hacking a random device
![]() | Still need to find the voltage this thing runs on, I think it's at least 30v [link] [comments] |
Just finished writing automatically updated KiCad library with all the basic/prefered JLCPCB parts and 3D models - thought I'd share!
![]() | submitted by /u/CD_FER [link] [comments] |
Found a bunch of Radio Shack parts from 40+ years ago that I never used
![]() | submitted by /u/Rocky_Mountain_Way [link] [comments] |
Nixie tubes are so unique and beautiful. I'm almost 5 years into running this clock and my goal is 20 on the original tubes.
![]() | submitted by /u/lxl_Arctic_lxl [link] [comments] |
Pages
