Українською
  In English
ELE Times


Ensuring Seamless Connectivity: Guide to In-Building Wireless Systems and Distributed Antenna Systems
Courtesy: Anritsu
In our increasingly mobile-dependent world, the need for reliable wireless coverage and capacity inside buildings has become paramount. This blog will delve into the concept of In-Building Wireless (IBW) systems, their significance, and the various architectures and technologies used to provide enhanced network coverage and capacity indoors. Understanding these systems is crucial as they are the backbone of our modern communication, enabling seamless connectivity in our homes, offices, and public spaces.
The demand for IBW systems becomes apparent when the existing macro network fails to meet the need for coverage and capacity within buildings adequately. However, challenges such as building structure, low emissivity glass, and RF barriers created by adjacent buildings can lead to subpar coverage. Overcoming these challenges is a testament to the importance and complexity of IBW systems, especially in densely populated urban areas and high-density venues like stadiums and convention centers.
The funding and ownership models for IBW systems are diverse, reflecting the various benefits derived from enhanced wireless services. Operators sometimes negotiate leases with building owners, taking on the costs of designing, installing, and maintaining the IBW system in exchange for exclusive coverage rights. In other cases, building owners are mandated by regulations to provide public safety coverage and cover the entire cost of the IBW system. Cost-sharing is arranged in venues like shopping centers and stadiums, where both the building owner and mobile operators benefit, showcasing the adaptability and flexibility of IBW solutions.
IBW solutions are not one-size-fits-all. They can be categorized into three main architectures, each with its own unique features and benefits: Distributed Antenna Systems (DAS), Distributed Radio Systems (DRS), and Distributed Small Cells (DSC). The choice of architecture is a critical decision, depending on various factors, including coverage objectives, budget, venue size, number of operators, and required technologies, emphasizing the importance of this selection process.
DAS is the most common method used to achieve IBW coverage and capacity. It involves distributing signals from RF sources throughout the venue using passive components like coaxial cables, splitters, and directional couplers. DAS can accept RF inputs from various signal sources, making it technology-neutral and suitable for multi-operator applications. There are different types of DAS, each with its own unique characteristics: passive DAS, active DAS, hybrid DAS, and digital DAS, each with its strengths and weaknesses.
Passive DASPassive DAS uses only passive components to distribute signals throughout the venue. It relies on coaxial cables, splitters, and directional couplers to divide the signal and achieve the desired signal level at each antenna. Signal sources for passive DAS can include repeaters, bi-directional amplifiers (BDAs), and small cells. Passive DAS is cost-effective, reliable, and suitable for multi-operator systems. However, it can be challenging to modify after installation and may encounter passive intermodulation (PIM) issues.
Active DASActive DAS involves converting signals to light and distributing them over fiber optic cables to radio units distributed throughout the venue. It offers greater flexibility in modifying sectorization and fine-tuning radiated power levels. Active DAS requires higher upfront costs but provides benefits such as easier cable routing, longer battery life for mobile users, and the ability to monitor system performance. It is suitable for large venues and multi-operator applications.
Hybrid DASHybrid DAS combines active and passive components to mitigate costs while maintaining performance. It uses active components for long-distance signal transport and passive components for signal distribution within each zone. Hybrid DAS provides a balance between cost, performance, and maintainability, making it suitable for multi-operator systems serving large and complex venues.
Digital DASDigital DAS utilizes radios manufactured by the DAS equipment provider and can accept RF inputs from various network equipment manufacturers. It uses digital transport protocols to distribute signals and offers dynamic resource allocation within the venue. Digital DAS supports centralized radio access network (C-RAN) and enables the backhaul of IP traffic. It provides flexibility and scalability, making it suitable for venues with changing coverage requirements.
ConclusionIn-building wireless systems are crucial in providing reliable coverage and capacity inside buildings. The choice of IBW architecture depends on various factors, including coverage objectives, budget, and venue size. Passive DAS, active DAS, hybrid DAS, and digital DAS each have their strengths and weaknesses, and the selection should be based on the specific requirements of the application. As mobile devices evolve and data demands increase, IBW systems will continue to play a vital role in meeting the growing demand for indoor wireless connectivity.
The post Ensuring Seamless Connectivity: Guide to In-Building Wireless Systems and Distributed Antenna Systems appeared first on ELE Times.
Applied Materials Unveils Chip Wiring Innovations for More Energy-Efficient Computing
- Industry’s first use of ruthenium in high-volume production enables copper chip wiring to be scaled to the 2nm node and beyond and reduces resistance by as much as 25%
- New enhanced low-k dielectric material reduces chip capacitance and strengthens logic and DRAM chips for 3D stacking
Applied Materials, Inc. introduced materials engineering innovations designed to increase the performance-per-watt of computer systems by enabling copper wiring to scale to the 2nm logic node and beyond.
“The AI era needs more energy-efficient computing, and chip wiring and stacking are critical to performance and power consumption,” said Dr. Prabu Raja, President of the Semiconductor Products Group at Applied Materials. “Applied’s newest integrated materials solution enables the industry to scale low-resistance copper wiring to the emerging angstrom nodes, while our latest low-k dielectric material simultaneously reduces capacitance and strengthens chips to take 3D stacking to new heights.”
Overcoming the Physics Challenges of Classic Moore’s Law ScalingToday’s most advanced logic chips can contain tens of billions of transistors connected by more than 60 miles of microscopic copper wiring. Each layer of a chip’s wiring begins with a thin film of dielectric material, which is etched to create channels that are filled with copper. Low-k dielectrics and copper have been the industry’s workhorse wiring combination for decades, allowing chipmakers to deliver improvements in scaling, performance and power-efficiency with each generation.
However, as the industry scales to 2nm and below, thinner dielectric material renders chips mechanically weaker, and narrowing the copper wires creates steep increases in electrical resistance that can reduce chip performance and increase power consumption.
Enhanced Low-k Dielectric Reduces Interconnect Resistance and Strengthens Chips for 3D StackingApplied’s Black Diamond material has led the industry for decades, surrounding copper wires with a low-dielectric-constant – or “k-value” – film engineered to reduce the buildup of electrical charges that increase power consumption and cause interference between electrical signals.
Applied today introduced an enhanced version of Black Diamond, the latest in the company’s Producer Black Diamond PECVD* family. This new material reduces the minimum k-value to enable scaling to 2nm and below, while offering increased mechanical strength which is becoming critical as chipmakers and systems companies take 3D logic and memory stacking to new heights.
The latest Black Diamond technology is being adopted by all leading logic and DRAM chipmakers.
New Binary Metal Liner Enables Ultrathin Copper WiresTo scale chip wiring, chipmakers etch each layer of low-k film to create trenches, then deposit a barrier layer that prevents copper from migrating into the chip and creating yield issues. The barrier is then coated with a liner that ensures adhesion during the final copper reflow deposition sequence, which slowly fills the remaining volume with copper.
As chipmakers further scale the wiring, the barrier and liner take up a larger percentage of the volume intended for wiring, and it becomes physically impossible to create low-resistance, void-free copper wiring in the remaining space.
Today, Applied Materials publicly introduced its latest IMS (Integrated Materials Solution) which combines six different technologies in one high-vacuum system, including an industry-first combination of materials that enables chipmakers to scale copper wiring to the 2nm node and beyond. The solution is a binary metal combination of ruthenium and cobalt (RuCo), which simultaneously reduces the thickness of the liner by 33 percent to 2nm, produces better surface properties for void-free copper reflow, and reduces electrical line resistance by up to 25 percent to improve chip performance and power consumption.
The new Applied Endura Copper Barrier Seed IMS with Volta Ruthenium CVD is being adopted by all leading logic chipmakers and began shipping to customers at the 3nm node. An animation of the technology can be viewed here.
Customer Comments“While advances in patterning are driving continued device scaling, critical challenges remain in other areas including interconnect wiring resistance, capacitance and reliability,” said Sunjung Kim, VP & Head of Foundry Development Team at Samsung Electronics. “To help overcome these challenges, Samsung is adopting multiple materials engineering innovations that extend the benefits of scaling to the most advanced nodes.”
“The semiconductor industry must deliver dramatic improvements in energy-efficient performance to enable sustainable growth in AI computing,” said Dr. Y.J. Mii, Executive Vice President and Co-Chief Operating Officer at TSMC. “New materials that reduce interconnect resistance will play an important role in the semiconductor industry, alongside other innovations to improve overall system performance and power.”
A Growing Wiring OpportunityApplied is the industry leader in chip wiring process technologies. From the 7nm node to the 3nm node, interconnect wiring steps have approximately tripled, increasing Applied’s served available market opportunity in wiring by more than $1 billion per 100,000 wafer starts per month (100K WSPM) of greenfield capacity, to approximately $6 billion. Looking ahead, the introduction of backside power delivery is expected to increase Applied’s wiring opportunity by another $1 billion per 100K WSPM, to approximately $7 billion.
The new chip wiring products, along with other materials engineering innovations for making future AI chips, will be discussed at Applied’s SEMICON West 2024 Technology Breakfast. The presentation and other materials from the event will be available on the Applied Materials website at: https://ir.appliedmaterials.com on Tuesday, July 9, 2024 at approximately 9:00 a.m. ET / 6:00 a.m. PT.
*PECVD = Plasma-Enhanced Chemical Vapor Deposition
*CVD = Chemical Vapor Deposition
Forward-Looking StatementsThis press release contains forward-looking statements, including those regarding anticipated benefits of our new products and technologies, expected growth and trends in our businesses and markets, industry outlooks and demand drivers, technology transitions, and other statements that are not historical facts. These statements and their underlying assumptions are subject to risks and uncertainties and are not guarantees of future performance. Factors that could cause actual results to differ materially from those expressed or implied by such statements include, without limitation: failure to realize anticipated benefits of our new products and technologies; the level of demand for semiconductors and for our products and technologies; customers’ technology and capacity requirements; the introduction of new and innovative technologies, and the timing of technology transitions; market acceptance of existing and newly developed products; the ability to obtain and protect intellectual property rights in technologies; our ability to ensure compliance with applicable law, rules and regulations; and other risks and uncertainties described in our SEC filings, including our recent Forms 10-Q and 8-K. All forward-looking statements are based on management’s current estimates, projections and assumptions, and we assume no obligation to update them.
The post Applied Materials Unveils Chip Wiring Innovations for More Energy-Efficient Computing appeared first on ELE Times.
electronica China 2024: 3 questions and a slew of solutions
Author: STMicroelectronics
What questions will ST answer at electronica China 2024? Too often, attendees don’t take the time to step back and ask what challenges they would need to solve or think of the answers they should seek. It’s easy to get caught up in the event’s excitement and the flood of information. That’s why we decided to highlight some of the questions our readers may have and provide answers. Hence, even if members of our community can’t physically attend this event, they will still get tools and ideas that can help them find innovations and efficiency.
What would it take to build an ST car? A powertrain domain controller!Attendees at electronica China 2024 will probably be surprised when they see the ST car, a small mock-up electric vehicle. The demo aims to show a modern approach to domain controllers by grouping multiple systems under one host MCU. Thanks to its multiple cores and virtualization capabilities, the Stellar P3 can support mid-level integrated electrification applications, while the Stellar P6 could run even more highly integrated electrification systems. We also increased the battery management system from a previous demo to support 800 V and worked to create an ASIL-D-ready system. In a nutshell, we show how ST solution advantages can help design teams shorten the development cycle and gain market shares.
In our demo car, the host MCU runs the onboard charger and DC-DC converter, the vehicle control unit, which is responsible for the relays, pumps, charger-lock, and more, the battery management system, and the inverter. Additionally, we provide solutions for engine management systems (EMS), transmission control units (TCU), thermal management systems, and more. Integrators can focus on the application instead of worrying about how the electronics will work together. The platform we are showing is continuously evolving, and we’ll release a new version before the end of the year. In the meantime, we continue to show how car makers can take advantage of the computational throughput and safety features of a device like the Stellar P.
As readers of the blog will remember from our power liftgate demo, the industry is looking into consolidating resources. It is one of the simplest ways to bring new features to mainstream vehicles. It simplifies development and helps reduce costs by sourcing more components from the same company, which significantly helps with overall pricing. The ST mock-up vehicle is another example of how ST is helping makers jump on the bandwagon. We also try to make critical technologies more accessible, like over-the-air updates, thanks to our phase-change memory, which is capable of increasing its capacity to enable flashing a new firmware without requiring hardware partitioning like A/B memory banks.
How do you promote sustainability? With integrated hybrid photovoltaic, energy storage, and charging solutions!Too often, cities know they need to implement new energy strategies that combine solar panels, energy storage, and charging solutions. Moreover, car makers must learn to utilize these new systems, which can lead to a lack of cohesion or slow adoption. Consequently, ST is featuring an energy storage and management solution demo at electronica China 2024 that shows how our devices can help deliver power across infrastructures. It uses our third-generation SiC MOSFETS, enhancement mode PowerGaN transistors, high-performance MCUs, galvanic isolation gate drivers, and more to control and protect the entire system. Put simply, it’s about achieving higher efficiency, better stability, and greater reliability than the industry traditionally offers today.
E-meterIt starts with a power line and hybrid platform for e-meters that uses the STM32WL3, which includes two radios and one ultra-low power wireless MCU. The board also comes with the ST8500 programmable powerline modem and the STLD1 driver.
Micro-inverterMany charging solutions rely on solar energy to further improve their environmental impact. Hence, an efficient micro-inverter that transforms what solar panels capture is critical. That’s why we are featuring a micro-inverter powered by our MASTERGAN1L solution, which is key to such a small factor thanks to its wide bandgap. Additionally, the module runs on an STM32G4, thanks to its high-resolution timers and high-precision ADCs.
Main inverterTo ensure greater efficiency when converting solar energy and distributing it, ST developed an inverter powered by silicon carbide: the SCT070W120G3-4AG and SCT055W65G3-4AG. After 25 years of innovations, ST can offer a 15kW three-phase three-level Active Front End bidirectional PFC converter. We even provide design tools like STPOWER Studio to help engineers design their PCBs quickly.
Battery chargingTo send power from the charger to the battery, we offer a couple of systems, both running on an STM32G4. We even provide the STGAP2SICS for SiC MOSFETs, which implements an under-voltage lockout (UVLO) to ensure the safe, stable, and efficient operation of the entire system.
Battery managementFinally, to manage the energy stored in the car’s batteries, we offer the AEK-POW-BMS63EN, a development platform for the L9963E that makes battery management solutions vastly more accessible. Indeed, thanks to its battery holder, its ability to daisy chain up to 31 nodes in minutes, its board dedicated to isolation, and software that helps take advantage of its features, engineers can significantly reduce their time to market.
How do you detect the orientation of a cup and its liquid level in a short time? Smart Cup Detection
It’s a complex problem because it requires fast computing, vision, and other sensors to determine where the cup is and how much liquid is in it. Or, one could just use the VL53L7CH or VL53L8CH time-of-flight sensors from ST. That’s what we will show at electronica China 2024 with a demo of a simulated coffee machine that can measure 64 different zones, represent the data in a compact normalized histogram, and enable the creation of a machine learning algorithm capable of detecting if the cup is upside down, off-center, absent, its height, and how much liquid it contains.
The only significant difference between the VL53L7CH and VL53L8CH is that the former has greater coverage and field of view for applications that monitor a larger area. The demo uses an X-NUCLEO-53L8A1 development board and a 3D-printed housing shaped like a coffee machine. Put simply, it showcases how integrators can create a complex detection system with few components and processing requirements, making this technology highly accessible.
The post electronica China 2024: 3 questions and a slew of solutions appeared first on ELE Times.
Electrification and ADAS: Fuelling a $14.3 Billion Sensor Market by 2029
Since the 20th-century introduction of semiconductors in automobiles, sensing technologies have steadily expanded. Initially aimed at enhancing powertrain efficiency, sensors like ABS, ESC, and airbags have become indispensable due to increasing safety priorities. Technological advancements have transitioned these innovations from luxury vehicles to mid-range and budget models, creating a multi-billion-unit industry. In 2023, 6.5 billion automotive sensors were shipped globally, generating $9.3 billion in revenue. With a 7% revenue CAGR from 2023 to 2029, the market is expected to reach $14.3 billion, with 8.8 billion sensors shipped worldwide. The automotive industry is poised for massive transformations in all four car domains:
- Powertrain and Electrification: The industry is shifting from internal combustion engine (ICE) cars, which constituted 91% of production in 2019, to electrified cars, expected to represent 43% of production by 2029. The Powertrain and Electrification sensor market will exceed $1.4 billion by 2029, growing at a 3% CAGR from 2023 to 2029. Electrification will drive critical changes in the sensor landscape, creating new applications and phasing out others.
- ADAS and Safety: Cars are becoming more intelligent, with sensors like cameras, LiDARs, and radars enabling cars to understand and react to their environment. The ADAS and Safety segment is projected to generate $8 billion in revenue by 2029, making it the largest segment.
- Infotainment and Telematics: This sector is driven by increased functionality and in-cabin comfort, with customers demanding better entertainment options. It is projected to achieve a 7% CAGR from 2023 to 2029, surpassing $2.9 billion by 2029.
- Chassis and Others: To enhance passenger safety, send valuable data to the ADAS computing unit, and eliminate hydraulic parts, sensor volumes are expected to grow at a 3% CAGR from 2023 to 2029. Revenue from this segment is expected to reach almost $1.9 billion by 2029.
Advancements in ADAS, autonomous driving, and electrification, alongside widespread sensor adoption, are set to transform automotive sensor technology. This will lead to substantial industry reorganization and supply chain changes, necessitating strategic business planning and partnerships to capitalize on emerging opportunities.
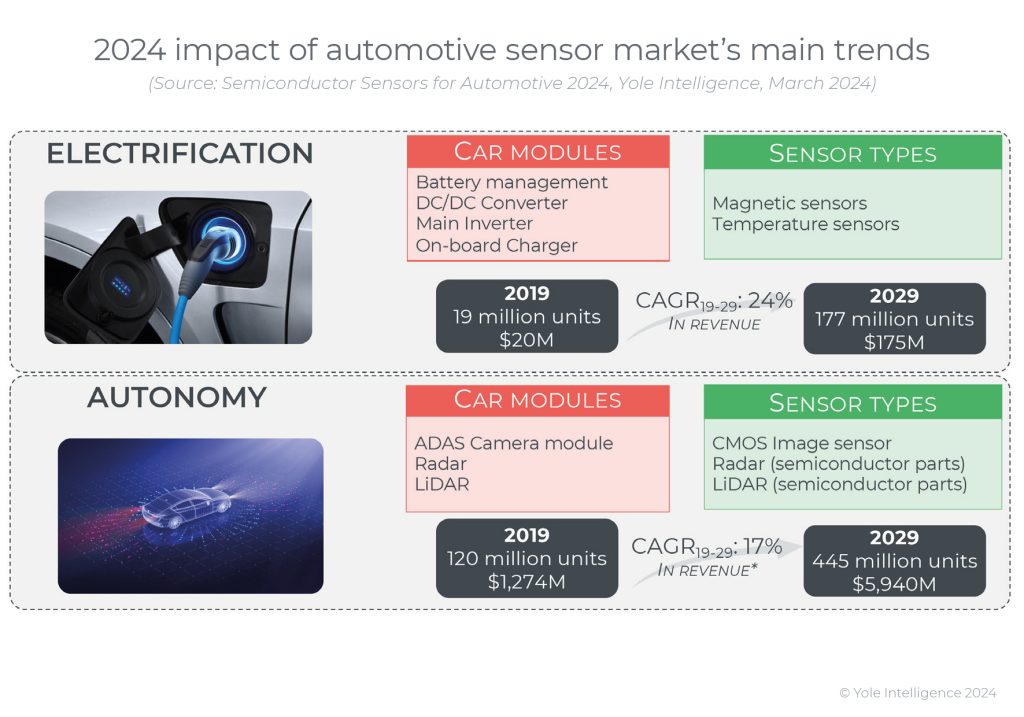
The growth or decline of sensor manufacturers will depend on their strategic positioning within the market as vehicles increasingly embrace electrification and advanced driver assistance systems (ADAS) and safety features.
- ICE Applications: Suppliers of sensors used in traditional ICE applications (e.g., engine pressure sensing, emission control, engine management systems) will face declining demand. This impact will become more pronounced by the decade’s end, especially with the 2035 ban on new ICE cars in Europe.
- New Prospects: Suppliers of MEMS pressure sensors and gas & particle sensors, such as Bosch, TE Connectivity, NXP, and Infineon, will find new opportunities in thermal runaway and CO2 sensing applications. Preventing thermal runaway at the battery level is critical for OEMs, and CO2 sensing is becoming a key in-cabin comfort feature.
- Magnetic and Temperature Sensors: Suppliers like Allegro Microsystems, Melexis, Infineon, and Sensirion will see significant opportunities. Monitoring current and temperature parameters is essential for understanding EV performance, leading to robust revenue growth for these players.
The increased presence of ADAS and safety components will primarily impact companies involved in image, radar, and LiDAR sensors.
- Safety Applications: As airbags and ESP become standard in nearly all vehicles, the expansion of MEMS accelerometers and inertial combo/IMUs will depend on vehicle sales growth. This trend is expected to generate substantial sales volumes for leading players such as Bosch, Murata, NXP, and STMicroelectronics.
- Image and Radar Sensors: These sensors are projected to experience significant growth due to the expanding adoption of Autonomous Emergency Braking (AEB) features in semi-autonomous vehicles.
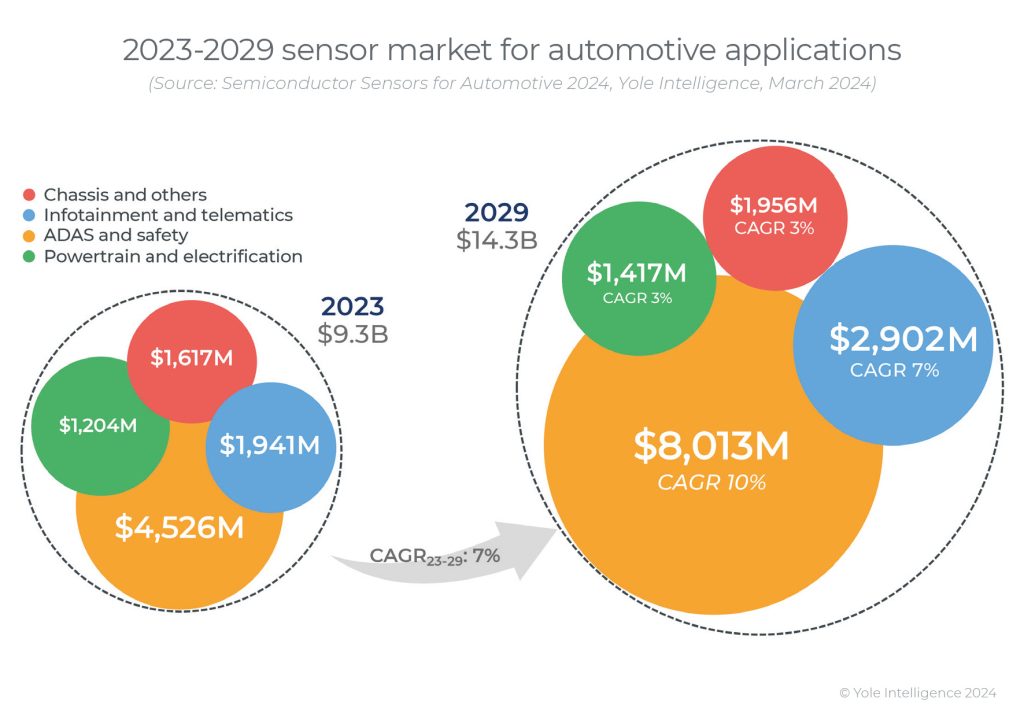
Though electrification and ADAS & safety are primary drivers, technology will evolve in all car domains.
- Powertrain and Electrification: Increasing battery capacities and advancing 800V batteries will drive the adoption of magnetic (isolated) technologies for current sensing in components like OBC, DC/DC converters, inverters, and power modules. Magnetic sensors will replace shunt sensors due to their lack of galvanic isolation. Additionally, maintaining optimal battery temperature is crucial for performance, safety, and lifespan. Magnetic sensors on thermal valves will regulate cooling or heating fluids more precisely, keeping sensor content per BEV around $15 per car.
- ADAS and Safety: CMOS image sensors will evolve to increase frame rates, dynamic range, and temperature resistance, meeting the needs of Tier-1s and OEMs. Radar sensors will advance with 4D imaging radar achieving a 1° angular resolution. LiDAR technology will improve in range, transitioning from avalanche photodiodes to more sensitive SPADs and SiPMs. The introduction of FMCW LiDAR by the end of the decade could change the technology landscape, with semiconductor content for ADAS and Safety sensors per car growing from $51 in 2023 to $82 in 2029.
- Infotainment and Telematics: Integration of sensors for comfort features will increase. MEMS sensors will improve phone call quality and enable noise-canceling functionalities. Capacitive sensors will replace physical buttons, and radar technology will be used for occupant monitoring. Environmental sensors will enhance in-cabin air quality.
- Chassis and Others: Innovations will focus on brake and drive-by-wire technologies to eliminate hydraulic components. Magnetic sensors will be essential for pedal position sensing and motor position monitoring. Intelligent TPMS modules will measure tire pressure and provide insights into tire quality and vehicle load. Additionally, applications for monitoring driver’s seating position and making slight adjustments during extended journeys will emerge.
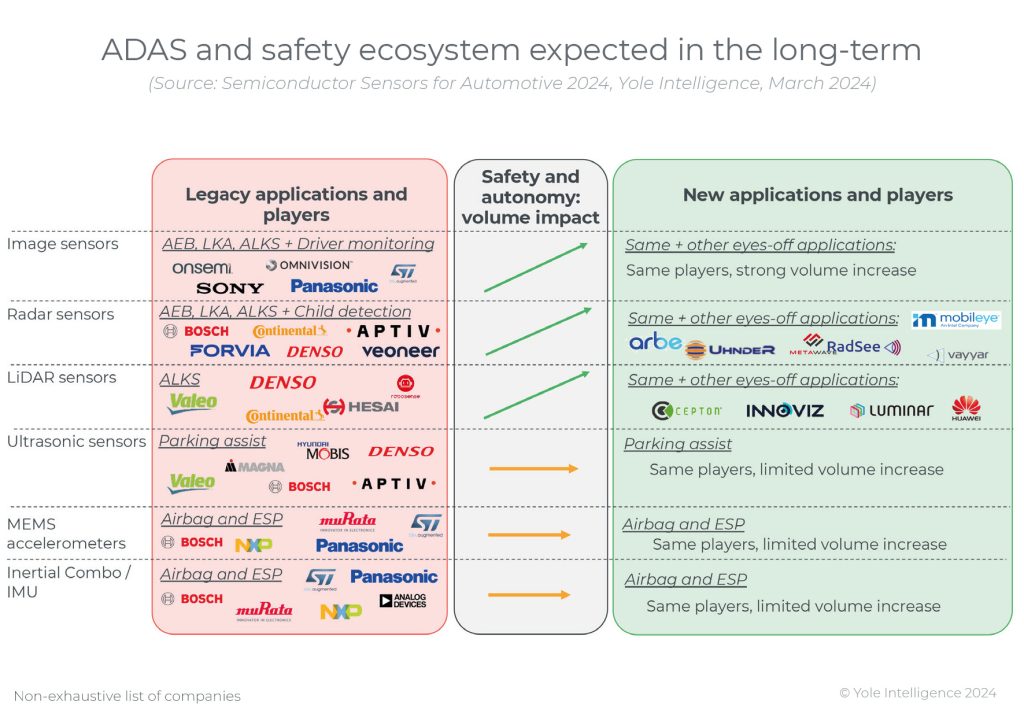
Citations from Yole Group
The post Electrification and ADAS: Fuelling a $14.3 Billion Sensor Market by 2029 appeared first on ELE Times.
Securing the Automotive Intelligent Edge
Courtesy: Analog Devices
Today’s automobiles are becoming increasingly sophisticated, especially with the advancement of self-driving technology. This includes features such as automated steering, acceleration, and braking, as well as convenient options like touchscreen controls and voice commands. Cars are now capable of performing tasks autonomously, such as activating high beams, parking themselves, detecting blind spots, and applying brakes to prevent accidents. These capabilities are made possible by electronic control units (ECUs) that connect to various parts of the vehicle, supporting systems like advanced driver assistance, power management, infotainment, and security. These parts must meet strict manufacturer specifications to ensure safety and performance. However, how does the automotive company ensure these requirements are being met?
One approach is through car part pairing, which involves cryptographic authentication and association between different vehicle subsystems to establish mutual trust. In simpler terms, car part pairing is a unique association of a part with a vehicle. So, trust in automotive components, such as sensors and actuators, requires OEM approval, provability, and secure control throughout their lifecycle.
You may wonder, what are the benefits of car part pairing for the automotive company? Let’s explore a few in this blog.
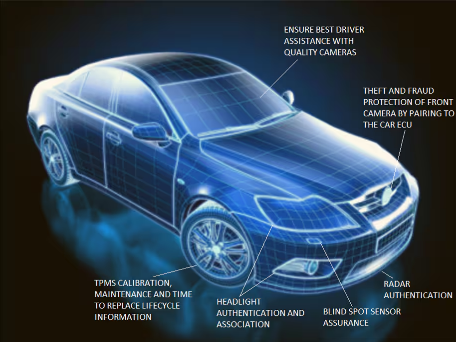
The primary benefit of pairing is to provide strong cryptographic identification and authentication of car parts. By securely linking specific car parts to specific vehicles, manufacturers can ensure that only authorized parts are used, improving safety and preventing fraud, theft, and counterfeiting. Risks are mitigated through this authentication scheme, as any replacement part must now be authentic and valid, eliminating counterfeit or stolen parts.
Another benefit of pairing is the ability to store and attest to the lifecycle of a car part, including calibration, manufacturing steps, maintenance, mounting, calibration, decommissioning, and relevant traceability information. Cryptographic methods using digital signatures provide formal proof of the car part’s state. This additional information can be used by the car’s ECUs to manage otherwise authentic parts, such as rejecting improperly calibrated OEM ADAS cameras or parts mounted into another car without proper authorization. This attestation of a part’s lifecycle reduces the risk of using invalid parts, even if they are authentic, provided the ECU is secure enough to prevent bypassing part verification.
Ensuring secure lifecycle data is crucial for trust. Tampering with this information could allow refurbishment of worn-out or malfunctioning parts, posing safety risks or using stolen parts. By employing cryptographic-based access control, manufacturers can ensure only authorized parties can modify the car part’s lifecycle information memory and other data used to bind the part to an ECU. Approved OEM dealers can then replace a part, associate it with the car chassis, and perform necessary calibrations.
Applying the BenefitsOne of the notable benefits lies in the application of cryptographic methods to establish the authenticity of a car’s installed ADAS camera in relation to the car’s ECU. This is commonly achieved through the utilization of the Elliptic Curve Digital Signature Algorithm (ECDSA) for challenge-response authentication with prior certificate verification.
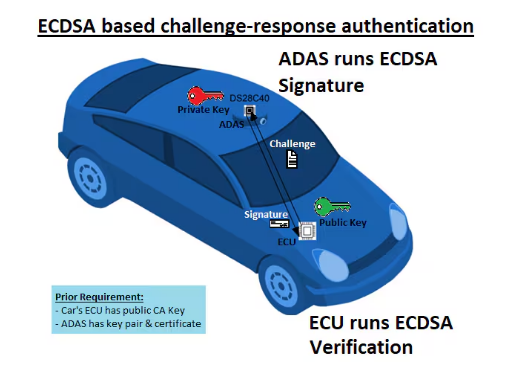
To provide clarity, challenge-response authentication involves the following steps:
- Challenge Initiation:The ECU verifier initiates the process by sending a “challenge” (a randomly generated number) to the ADAS prover.
- Response Generation:The ADAS prover, using a private key, digitally signs the received random number, generating a “response.” Additional information, such as unique identification data, life cycle data and calibration data, may be concatenated with the random number for contextualization.
- Transmission of Response: The ADAS prover then transmits the generated “response” to the ECU.
- Verification Process:The ECU verifier runs a verify, with the public key, using the prover’s identification data and ECDSA signature response to ascertain authenticity. Importantly, this authentication process is bidirectional, capable of being performed in both directions.
This framework ensures robust authentication, affirming the integrity of the ADAS camera’s association (i.e., proving it is paired) with the ECU. Subsequently, cryptographic methods can prove a car’s mounted ADAS camera is paired with proper car’s ECU.
All these benefits listed in this blog can be assured with the DS28C40 Automotive I2C Authenticator IC. Analog Devices offers both the software and experience to implement car part pairing, contributing to building safer automobiles.
The post Securing the Automotive Intelligent Edge appeared first on ELE Times.
EMITE and Rohde & Schwarz join forces to support OTA measurements on Wi-Fi 7 and 5G RedCap
EMITE and Rohde & Schwarz continue their collaboration to enhance EMITE’s Over-the-Air (OTA) measurement solutions by fully integrating the latest test capabilities of the R&S CMX500 multi-technology, multi-channel one-box signaling tester. The integration enables besides support of LTE, FR1 and FR2 also full compliance with the cutting-edge WLAN standard IEEE 802.11be as well as 5G RedCap.
EMITE’s state-of-the-art over-the-air chambers can now be combined with the latest sophisticated testing features of the R&S CMX500 one-box signaling tester from Rohde & Schwarz. The radio communication tester offers a wide range of device testing capabilities, supporting cellular technologies such as LTE, 5G NR FR1 frequency range up to 8 GHz and FR2 millimeter wave frequency range up to 50 GHz, including the latest 5G RedCap technology. It also supports non-cellular technologies, including the latest Wi-Fi 7, all in a single instrument. As a consequence of this collaboration, EMITE’s customers obtain a comprehensive environment for accurately assessing the performance of Wi-Fi 7 as well as 5G Red Cap devices.
EMITE’s enhanced OTA chambers are fully equipped to handle the rigorous testing requirements of current and future wireless technologies. The next step of the collaboration is looking at integrating test capabilities for NB-IoT NTN and NR NTN, which are already covered by the R&S CMW500 and R&S CMX500.
The IEEE 802.11be standard, Wi-Fi 7, is the next generation of Wi-Fi technology, designed for extremely high data throughput that far surpass its predecessor, the current IEEE 802.11ax (Wi-Fi 6/6E). With tens of gigabits of data per second and low latency, it meets the growing demand for ultra-high-definition video streaming, as well as immersive virtual reality and augmented reality applications.
RedCap is a 5G technology defined in 3GPP Release 17. As a reduced-functionality version of 5G, it has significantly lower cost compared to 5G eMBB. It is characterized by mid-range data throughput, low power consumption, low complexity and the ability to support a large number of devices. This makes it particularly attractive for IoT applications.
David A. Sánchez-Hernández, CEO at EMITE, said: “EMITE is committed to quality and customer satisfaction. Therefore, we are excited to upgrade our testing chambers with the R&S CMX500 enabling our customers to extend capabilities to include the IEEE 802.11be standard and Red Cap devices. This partnership underscores our dedication to delivering superior testing solutions that meet the evolving demands of the telecommunications sector.”
Christoph Pointner, Senior Vice President of Mobile Radio Testers at Rohde & Schwarz, said: “We are happy to bring our collaboration with EMITE to the next level. EMITE’s customers can confidently deploy their devices for the latest technologies, knowing their products have been rigorously tested to meet the highest standards of reliability and efficiency, made possible through the integration of our tried-and-tested R&S CMX500 one-box signaling tester into the EMITE OTA chambers portfolio.”
For more information on the R&S CMX500, visit: www.rohde-schwarz.com/product/cmx500 . For more information on EMITE, visit: www.emite-ing.com
The post EMITE and Rohde & Schwarz join forces to support OTA measurements on Wi-Fi 7 and 5G RedCap appeared first on ELE Times.
AAEON’s MAXER-2100 Inference Server Integrates Both Intel CPU and NVIDIA GPU Technologies
The MAXER-2100 illustrates AAEON’s commitment to innovation across client bases.
Leading provider of advanced AI solutions AAEON (Stock Code: 6579), has released the inaugural offering of its AI Inference Server product line, the MAXER-2100. The MAXER-2100 is a 2U Rackmount AI inference server powered by the Intel Core i9-13900 Processor, designed to meet high-performance computing needs.
The MAXER-2100 is also able to support both 12th and 13th Generation Intel Core LGA 1700 socket-type CPUs, up to 125W, and features an integrated NVIDIA GeForce RTX 4080 SUPER GPU. While the product’s default comes with the NVIDIA GeForce RTX 4080 SUPER, it is also compatible with and an NVIDIA-Certified Edge System for both the NVIDIA L4 Tensor Core and NVIDIA RTX 6000 Ada GPUs.
Given the MAXER-2100 is equipped with both a high-performance CPU and industry-leading GPU, a key feature highlighted by AAEON upon the product’s launch is its capacity to execute complex AI algorithms and datasets, process multiple high-definition video streams simultaneously, and utilize machine learning to refine large language models (LLMs) and inferencing models.
Given the need for latency-free operation in such areas, the MAXER-2100 offers up to 128GB of DDR5 system memory through dual-channel SODIMM slots. For storage, it includes an M.2 2280 M-Key for NVMe and two hot-swappable 2.5” SATA SSD bays with RAID support. The system also provides extensive functional expansion options, including one PCIe [x16] slot, an M.2 2230 E-Key for Wi-Fi, and an M.2 3042/3052 B-Key with a micro SIM slot.
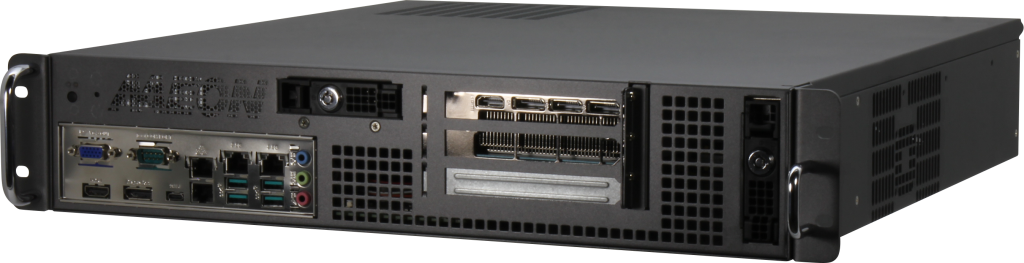
For peripheral connectivity, the server boasts a total of four RJ-45 ports, two running at 2.5GbE and two at 1GbE speed, along with four USB 3.2 Gen 2 ports running at 10Gbps. In terms of industrial communication options, the MAXER-2100 grants users RS-232/422/485 via a DB-9 port. Multiple display interfaces are available, thanks to HDMI 2.0, DP 1.4, and VGA ports, which leverage the exceptional graphic capability of the server’s NVIDIA GeForce RTX 4080 SUPER GPU.
Given the combined thermal output of its 1000W power supply, 125W CPU, integrated NVIDIA GeForce RTX 4080 SUPER GPU, and potential additional add-on cards, the MAXER-2100 is remarkably compact at just 17″ x 3.46″ x 17.6″ (431.8mm x 88mm x 448mm). This is made possible by a novel cooling architecture utilizing three fans, prioritizing airflow around the CPU and key chassis components. Fan placement within the MAXER-2100 chassis also serves to reduce system noise.
AAEON has indicated that the system caters to three primary user bases – edge computing clients, central management clients, and enterprise AI clients.
The first of these refers to organizations and businesses that require scalable, server-grade edge inferencing for applications such as automated optical inspection (AOI) and smart city solutions.
“The MAXER-2100 can be used to run multiple AI models across multiple high-definition video streams simultaneously, via either its onboard peripheral interfaces or scaled up via network port integration.” Associate Vice President of AAEON’s Smart Platform Division Alex Hsueh said. “Its high-performance CPU, powerful GPU, large memory capacity, and high-speed network interfaces make it well-equipped to handle the acquisition and processing of 50-100+ high definition video streams, making it an ideal solution for applications requiring real-time video analysis.” Hsueh added.
AAEON’s second target market is those seeking remote multi-device management functions, such as running diagnostics, deploying or refining AI models, or storing local data on edge devices. On the topic of the product’s suitability for such clients, Mr. Hsueh remarked, “With the MAXER-2100, our customers can utilize K8S, over-the-air, and out-of-band management to update and scale edge device operations across smart city, transportation, and enterprise AI applications, addressing key challenges faced by our customers when managing multiple AI workloads at the edge.”
For enterprise AI clients, AAEON indicates that by leveraging the MAXER-2100, companies can effectively harness their data to build and deploy advanced AI solutions powered by LLMs. This includes applications in natural language processing, content generation, and customer interaction automation. The key benefits that the MAXER-2100 brings to such setups are the security provided by data being stored and processed at the edge and the system’s ability to train and refine inference models during operations.
For more information and detailed specifications, please visit the MAXER-2100 product page, or contact your AAEON representative via the contact form on the AAEON website.
The post AAEON’s MAXER-2100 Inference Server Integrates Both Intel CPU and NVIDIA GPU Technologies appeared first on ELE Times.
Accenture Acquires Excelmax Technologies to Expand Silicon Design and Engineering Capabilities
Accenture has acquired Excelmax Technologies, a Bangalore, India-based semiconductor design services provider. The acquisition further enhances Accenture’s growing silicon design and engineering capabilities. Terms of the transaction were not disclosed.
Excelmax provides custom silicon solutions used in consumer devices, data centers, artificial intelligence (AI) and computational platforms that enable edge AI deployments, to clients in the automotive, telecommunications and high-tech industries.
The semiconductor market is experiencing a surge in demand for silicon design engineering, driven by the proliferation of data centers and the increasing use of AI and edge computing. This is further propelled by the growing consumer appetite for electronics, which is driving new investments in the chip design space.
“With the rapid evolution of new technologies like generative AI and the growth of connected products, more intricate, specialized chips with enhanced performance and efficiency are required,” said Karthik Narain, group chief executive—Technology at Accenture. “Our acquisition of Excelmax enhances our expertise across every aspect of silicon design and development—from concept to production—so we can help our clients fuel innovation and drive growth.”
Mahesh Zurale, global lead – Advanced Technology Centers Global Network, Accenture said, “Accenture’s acquisition of Excelmax Technologies brings approximately 450 highly skilled silicon professionals to our Advanced Technology Centers in India. With the global demand for silicon solutions on the rise, India is becoming a hotbed for chip design. Growing our skilled talent in the country across physical and RTL design, verification, emulation, and firmware engineering will help us expand our capabilities in the silicon design space and accelerate innovation for our world-wide clients.”
Founded in 2019, Excelmax brings comprehensive semiconductor solutions from high level design to detailed physical layout ready for manufacturing, and full turnkey execution. The company adds approximately 450 professionals to Accenture in key areas such as emulation, automotive, physical design, analog, logic design and verification, expanding Accenture’s ability to help global clients accelerate edge computing innovation.
“Our focus has always been on developing the best talent to deliver tailor-made solutions for our global clients that help them build and maintain competitive advantage,” said Shekhar Patil, founder & CEO, Excelmax Technologies. “Joining Accenture enables us to remain at the forefront of innovation, providing new and exciting opportunities for both our clients and our people.”
This acquisition follows the addition of XtremeEDA, an Ottawa, Canada-based silicon design services company, in 2022.
The post Accenture Acquires Excelmax Technologies to Expand Silicon Design and Engineering Capabilities appeared first on ELE Times.
National Instruments Celebrates 25 years of Technological Brilliance in India
In 2023, Emerson acquired NI and introduced its new Test & Measurement business group. Both NI and Emerson are committed to continuous innovation, operational excellence, and sustainability. Together, they are building on their legacy and leadership in software-connected, automated test and measurement systems. The team is dedicated to investing in industry-leading innovations, delivering differentiated solutions to global customers, and assisting engineers in solving the world’s toughest challenges. Their test automation technology is renowned for delivering exceptional accuracy, throughput, and reliability, from the laboratory to the manufacturing floor and beyond. With the industry’s most comprehensive software portfolio, modular hardware, systems, and services, NI’s software-connected approach revolutionizes how enterprises utilize test insights.
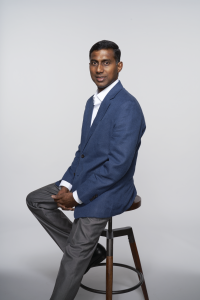
Rashi Bajpai, Sub-Editor at ELE Times spoke with Baskar Ceri, MD, NI India on completing 25 years in India.
This is an excerpt from the conversation.
- Having worked with National Instruments in diverse departments and roles, how has the journey been so far? Please throw some light on NI’s journey in India, and what according to you has been paramount in building a strong foothold in the country?
National Instruments (NI) has been instrumental in my career and it has been a fulfilling journey of growth and opportunity. I started my career at NI in Austin, USA and was part of the 3-member team that started NI operations in India growing the team and the revenue to multi-million dollars in India.
Since its establishment in India in 1999, NI has experienced significant expansion, now boasting a team of over 400 individuals spanning across its R&D and sales functions. Our journey has been guided by a strategic commitment to innovation, customer satisfaction, and adaptability. Paramount to our success has been our ability to understand and cater to the unique needs of the Indian market while contributing to global product development. This approach has enabled us to build a strong foothold in the country’s technology landscape.
2. What is NI’s vision for the Indian market at a time when the Indian vision is that of self-reliability and sustainability attaining momentum through policies like “Make in India”?
National Instruments (NI) envisions playing a pivotal role in India’s journey towards self-reliability and sustainability, our aim for the Indian market involves empowering local industries with cutting-edge test and measurement technology solutions that enhance manufacturing efficiency, product quality, and innovation. By leveraging our expertise in automation, standardization, and digital transformation, NI aims to support India’s ambition to become a global manufacturing hub, not only for electronics but also for semiconductors. We seek to enable Indian industries to produce world-class products while reducing dependency on imports and fostering economic growth. Through strategic partnerships, knowledge sharing, and investment in local talent, NI is committed to contributing to India’s vision of self-reliance and sustainability, driving progress and prosperity for the nation.
3. Tell us about your customer base in India and your product line that runs in the country.
In India, National Instruments (NI) serves a diverse customer base across various industries, including defense, aerospace, automotive, electronics, semiconductor, and energy sectors. Our products and solutions play a critical role in testing and measurement applications, ensuring the quality, reliability, and performance of a wide range of products.
Some of our notable customers in India include the Defense Research and Development Organization (DRDO), the Indian Space Research Organisation (ISRO), and various automotive and semiconductor companies. Our products are utilized in testing cellphones, satellites, missiles, automotive components, electronic devices, and more.
NI’s product line in India includes a comprehensive range of hardware, software, and systems for automated testing and measurement. This includes data acquisition systems, modular instruments, software platforms like LabVIEW, and integrated test systems tailored to specific industry needs. Our solutions enable customers to streamline testing processes, improve efficiency, and accelerate product development cycles, ultimately driving innovation and competitiveness in the Indian market.
4. Help us understand NI’s vision and goals for the next 25 years, and if you have a Plan of Action ready to implement
NI’s strategic vision is to be the global leader in software-connected automated electronic test and measurement systems. Since its establishment, NI has been at the forefront of innovation, offering a wide range of hardware, software, systems and services that enable engineers and researchers to design, prototype, and deploy cutting-edge technologies efficiently. NI’s mission is to equip engineers and enterprises with systems that accelerate productivity, innovation, and discovery.
NI embarked on its journey in India in 1999 with just three employees, quickly establishing its first R&D centre. Over the years, our team has expanded to over 400 employees, focusing on both R&D and sales, with headquarters in Bangalore and separate offices for sales and R&D.
Looking ahead to the next 25 years, after Emerson acquired NI and introduced its new Test & Measurement business group. NI and Emerson share commitments to continuous innovation, operational excellence, and sustainability. In this new chapter, we’re building on our legacy and leadership in software-connected, automated test and measurement systems. We remain focused on investing in industry-leading innovations, delivering differentiated solutions to our customers around the globe, and helping engineers solve the world’s toughest challenges.
The post National Instruments Celebrates 25 years of Technological Brilliance in India appeared first on ELE Times.
E-Fill Electric Honored as “Star EV Charging Station Manufacturer of the Year” at India EV 2024 Show & Conference
E-Fill Electric (EFEVChargingSolutionsPvtLtd.) proudly announces its prestigious recognition as the “Star EV Charging Station Manufacturer of the Year” at the India EV 2024 Expo and conference held at Chennai Trade Centre from June 29th to 30th, 2024. This award celebrates E-Fill Electric’s commitment to excellence and sustainability in the electric vehicle (EV) charging industry.
The India EV 2024 show and conference is a prominent platform that recognizes the outstanding contributions of industry leaders and trailblazers in advancing EV technology and infrastructure. E-Fill Electric’s dedication to innovation and quality in EV charging solutions has earned them this esteemed accolade, highlighting their significant impact on the EV ecosystem.
Mayank Jain, CEO, E-Fill Electric, expressed his gratitude and remarked, “Receiving the ‘Star EV Charging Station Manufacturer of the Year’ award is a testament to our team’s relentless efforts and dedication to revolutionizing sustainable transportation. We are honored to be recognized for our commitment to delivering cutting-edge EV charging solutions that meet the evolving needs of our customers and the EV Industry.”
Established in 2019, E-Fill Electric has emerged as a pioneer in EV charging solutions, catering to diverse stakeholders including individuals, businesses, CPOs, OEMs, and fleet operators. Their comprehensive range of AC and DC chargers, with power outputs ranging from 3.3kW to 240kW, ensures accessibility and efficiency across various applications.
Beyond manufacturing excellence, E-Fill Electric has established a robust franchise network spanning over 25 states and districts in India, empowering local entrepreneurs to participate in the EV charging infrastructure expansion. The company’s innovative approach extends to producing lithium-ion battery-powered electric three-wheelers, further solidifying their commitment to sustainable mobility solutions.
E-Fill Electric’s dedication to innovation is evident through their robust R&D efforts, which have resulted in securing over 7 patents. Their commitment to advancing electric vehicle (EV) technology underscores their leadership in driving the EV revolution forward.
For more information about E-Fill Electric and their award-winning EV charging solutions, please visit www.efillelectric.com.
The post E-Fill Electric Honored as “Star EV Charging Station Manufacturer of the Year” at India EV 2024 Show & Conference appeared first on ELE Times.
Revolutionizing NFC & RFID Reading Technology, the ultimate solution for seamless NFC & RFID data reading from your Android or iPhone devices.
REYAX Technology, a leading innovator in the IoT industry, is proud to announce the launch of its latest product, RYRR30D. This cutting-edge solution sets a new standard for connectivity, security, and convenience in the realm of NFC/RFID.
The RYRR30D is certified by Apple Wallet VAS & Google SmartTap pass, ensuring top-tier compatibility and security. Enjoy the convenience of NFC technology, superior to traditional RFID cards, with the added benefit of Apple ECP V1.0 compliance and OTA (Over-the-Air) programming for seamless updates.
The RYRR30D offers unparalleled versatility with support for UART and USB 2.0 interfaces. Configure IDs and Keys effortlessly and decrypt NFC pass data with ease. Plus, the RYRR30D supports ISO15693, ISO14443A, ISO14443B, and FeliCa, offering comprehensive RFID TAG reading functionality.
In comparison to traditional RFID cards, the NFC technology utilized in RYRR30D offers unparalleled convenience and heightened security. Its seamless integration with mobile platforms, coupled with enhanced connectivity and encryption, makes it the ideal choice for a wide range of applications: EV Charger Authentication, Concert or Event Ticket Validation, Digital Member Cards, Access authentication, Digital Coupons, Loyalty Cards, and Library Card, etc.
The post Revolutionizing NFC & RFID Reading Technology, the ultimate solution for seamless NFC & RFID data reading from your Android or iPhone devices. appeared first on ELE Times.
STMicroelectronics at electronica China 2024 :Cutting-edge technologies and innovative solutions for Automotive, Industrial, Personal Electronics and Cloud Infrastructure
STMicroelectronics, a global semiconductor leader serving customers across the spectrum of electronics applications, will exhibit at electronica China 2024(Booth E4.4600) on 8-10 July. Under the theme of “Our technology starts with You”, ST will showcase a comprehensive portfolio of innovative solutions across Automotive, Industrial, Personal Electronics and Cloud Infrastructure, featuring over 50 interactive demos and advanced solutions, each tailored to meet the evolving needs of our customers and the market.
Automotive: With over 30 years’ experience in automotive electronics, ST is a reliable and innovative partner for building the future of smart e-mobility. ST’s comprehensive solutions span the e-mobility value chain. From electric powertrains to digital vehicle platforms, ST technologies make electric transportation safer, cleaner, and more advanced. At electronica China, ST will demonstrate its complete automotive solutions by showcasing two electric-vehicle models. Visitors can explore ST’s wide range of applications for car electrification and digitalization, from Powertrain for Electric Vehicles (EV), Chassis and Safety, Body and Convenience, to Telematics and Infotainment.
Electrification is a major development trend in China’s automotive market, with prosperous growth in the NEV (new energy vehicle) market segment, accompanied by an increasing demand for convenient NEV charging solutions. ST’s latest automotive-charging solution integrates On Board Charger (OBC) and DC-DC converter built with STPOWER products enabling compatibility with different power levels like 6.6kW, 11kW, or even 22kW. It features ST’s Stellar E1 40nm microcontroller technology based on the ARM architecture, delivering high efficiency and reliability through high and flexible power computing cores with rich analog components and the highest functional safety level.
Another innovation ST will showcase at electronica China is the ADAS – Intelligent Front View Camera Solution, developed in collaboration with ST’s partners. This solution incorporates a range of ST’s technologies, including the SPC58NN automotive MCU and the L9396 System Basis Chip (SBC). The combination of an MCU and an SBC enables customers to meet the stringent safety requirements of ASIL-D when creating advanced ADAS products.
Industrial: With increasing concerns around climate change and energy costs, stakeholders, such as states, companies, and individuals, globally are prioritizing green-energy sustainable practices. ST is at the forefront of driving a greener future by developing sustainable solutions in a sustainable way. At electronica China, visitors are invited to witness a one-of-a-kind “energy-wall” that shows a comprehensive hybrid energy system. It illustrates the entire energy-conversion chain, from generation and storage to distribution and consumption, through various components and devices including PV solar panels, inverters, a Battery Management System (BMS), a bi-directional energy-storage system, and EV charging systems.
Reliable and efficient power is critical for datacenters supporting AI/cloud workloads. Using its cutting-edge technologies such as STPOWER Gen3 SiC MOSFETs and silicon transistors (i.e. high voltage MOSFETs and IGBTs), galvanic isolation gate drivers, high precision op amps, current sensing amplifiers, and high-performance MCUs, ST showcases solutions that aim to boost energy efficiency while cutting consumption and carbon emissions.
Arc Fault Circuit Interrupter (AFCI) has been widely applied in new energy industries such as photovoltaics and energy storage. At electronica China, ST will present its AFCI solution, which leverages edge-AI algorithms based on the STM32H7 and STM32G4 MCUs to detect arc faults in real time. Compared to traditional mechanistic algorithms, the AI algorithm integrated with the STM32 technology offers significantly higher accuracy and reduced false-alarm rates. The data training from a customer on-site and the upgrading of the STM32 AFCI 2.0 algorithm can lead to higher accuracy in the inference results.
Personal Electronics and Cloud Infrastructure: With the widespread adoption of NFC functionality in smartphones in recent years, an increasing number of brands are leveraging NFC to enhance consumer interaction and brand loyalty. High-end brands, high-value products, including luxury goods and artworks, require more secure NFC tag solutions to prevent counterfeiting. At this exhibition, ST also introduces its latest ST25TA-E NFC tag chip with state-of-the-art security features bringing innovation to the market with its on-chip ECDSA (Elliptic Curve Digital Signature Algorithm) capabilities and compatibility with Blockchain standards. Building on the Edge TruST25 digital-signature feature, the ST25TA-E includes an ECC cryptographic engine, providing a more secure digital-signature solution that can offer significant assistance in product-identity verification and digital twinning.
Showcased at electronica China, ST’s new third-generation duel full-scale MEMS pressure sensor comes in a sealed, cylindrical, surface-mountable package. The sensor is demoed in a solution that combines high precision and low power consumption to accurately measure water depth and altitude for IoT, sports, and wearable-device applications. It features a ceramic substrate that provides high resistance to liquid permeability and a robust potting gel, proven in automotive applications, to protect the internal circuitry.
Improving user experience is key to driving ST’s continuous innovation. Powered by ST FlightSense Time-of-Flight technology, a smart-cup detection shown at electronica China can detect and measure cups of various sizes, shapes, and materials, as well as their positions and the liquid level inside, thanks to the Compact Normalized Histograms feature. With this technology, users can use cups of any size and shape without worrying about spills due to poor fit.
A binocular 3D camera will be on show using one of our ST BrightSense global-shutter image sensors, which have just been launched on the open-market, to make this unique technology available to all, after having delivered > 1Bu image sensors to selected partners.
Presentations:
In addition to exciting demonstrations, ST’s experts will take the stage at electronica China to deliver 6 insightful presentations. These sessions will span a wide array of cutting-edge topics, offering deep dives into the latest trends and innovations that are shaping the future of technology:
- ST’s Edge AI Solutions
- ST’s Silicon Carbide MOSFET Technology Roadmap and Market Strategy in China
- ST motor control development ecosystem and system solution introduction
- The Solution of Intelligent Driving
- STM32 Solution in Digital Power and ESS
- Bluetooth LE Audio – Auracast with STM32WBA55
For an in-depth exploration of these captivating demonstrations, please visit ST’s booth (Booth E4.4600)at electronica China 2024.
The post STMicroelectronics at electronica China 2024 :Cutting-edge technologies and innovative solutions for Automotive, Industrial, Personal Electronics and Cloud Infrastructure appeared first on ELE Times.
AI to Transform Passive and Interconnect Design
Artificial Intelligence (AI) applications typically involve handling large datasets, necessitating multiple distributed CPUs and GPUs communicating in real time. This setup is a hallmark of high-performance computing (HPC) architectures. Routing high-speed digital signals between processing elements necessitates chip-to-board and board-to-board connectivity. To meet high-speed requirements, communication protocols and physical standards have been developed based on signal integrity standards, which also ensure interoperability among suppliers. Occasionally, non-standard connectors are used due to specific form-factor requirements or other mechanical constraints. In these cases, their suitability can be assessed by comparing their specifications with industry-standard parts.
AI Data Bandwidth in Signal IntegrityWhen considering signal integrity, bandwidth and impedance are crucial electrical characteristics. Pin count, materials used, and mounting methods are significant mechanical considerations that impact performance and reliability. As HPC systems consume more power, contact resistance becomes increasingly important for improving data centre power efficiency.
For CPU connectivity, solderless interfaces often take the form of land grid array (LGA) or pin grid array (PGA) packages. Intel pioneered the LGA, using it for almost all its CPUs. Processors not designed to be user-replaceable might use a ball grid array (BGA), which connects components to the printed circuit board using solder balls. This is common for GPUs and some CPUs. The rate of data transfer between memory and a processor is a key factor in system performance. The latest development in random access memory (RAM) is the shift from DDR4 to DDR5, with DDR4 supporting data rates up to 25.6 Gbps and DDR5 up to 38.4 Gbps.
This evolution influences chip interface design. The latest LGA 4677 IC sockets offer link bandwidth up to 128 Gbps, typically supporting 8-channel DDR5 memory. These tightly spaced connection points can carry up to 0.5 A, reflecting the power demands of modern high-performance processors. Dual inline memory modules (DIMM) DDR5 memory sockets now support up to 6.4 Gbps bandwidth, with mechanical designs that save space and improve airflow around components on the printed circuit board.
Connecting AI Beyond the BoardPCI Express
Most processor boards feature several PCI Express (PCIe) slots for connectors, with slot types ranging from x1 to x16. The largest slots are typically used for high-speed GPU connectivity. The PCIe protocol standard allows up to 32 bidirectional, low latency, serial communications “lanes,” each consisting of differential pairs for transmitting and receiving data. PCIe 6.0, announced in January 2022, doubles the bandwidth of its predecessor to 256 Gbps, operating at 32 GHz, although hardware availability is currently limited.
InfiniBand
InfiniBand, common in HPC clusters, offers high speed and low latency, with maximum link performance of 400 Gbps and support for many thousands of nodes in a subnet. It can use board form factor connections and supports both active and passive copper cabling, active optical cabling, and optical transceivers. InfiniBand is complementary to Fibre Channel and Ethernet protocols but offers higher performance and better I/O efficiency. Common connector types for high-speed applications include QSFP+, zQSFP+, microQSFP, and CXP.
Ethernet
High-speed Gigabit Ethernet is increasingly common in HPC, with main connector types including CFP, CFP2, CFP4, and CFP8. CFP stands for C-form factor pluggable, with CFP2/CFP4 offering up to 28 Gbps per lane and supporting 40 Gbps and 100 Gbps Ethernet CFP-compliant optical transceivers. CFP8 connectors support up to 400 Gbps connectivity with 16, and 25 Gbps lanes.
Fibre Channel
Fibre Channel, specific to storage area networks (SANs), is widely deployed in HPC environments, supporting both fibre and copper media. It offers low latency, high bandwidth, and high throughput, with current support of up to 128 Gbps and a roadmap to 1 Terabit Fiber Channel (TFC). Connector types range from traditional LC to zQSFP+ for the highest bandwidth connections.
SATA and SAS
Serial Attached Technology Attachment (SATA) and Serial Attached SCSI (SAS) are protocols designed for high-speed data transfer, primarily used to connect hard drives and solid-state storage devices within HPC clusters. Both have dedicated connector formats with internal and external variants. SAS is generally preferred for HPC due to its higher speed (up to 12 Gbps) but is more expensive than SATA. Often, the operating speed of the storage device limits data transfer rates.
Passive Components and Powering AI ProcessorsAs processing speed and data transfer rates increase, so do the demands on passive components. Powering AI processors in data centres require ferrite-cored inductors for EMI filtering in decentralized power architectures to carry tens of amps. Low DC resistance and low core losses are essential. Innovations like single-turn, flat wire ferrite inductors, designed for point-of-load power converters, are rated up to 53 A with maximum DC resistance ratings of just 0.32 mOhms, minimizing losses and heat dissipation.
High-performance processing necessitates high current and power rails with good voltage regulation and fast response to transients. Designers must consider frequency-dependent characteristics beyond capacitance and voltage ratings. Aluminium electrolytic capacitors, traditionally used for high capacitance values, are now often replaced by polymer dielectric and hybrid capacitors for their lower equivalent series resistance (ESR) and longer operating life.
The high power consumption of data centres has increased the voltage used in rack architectures from 12 V to 48 V for improved power efficiency. 48 V-rated aluminium polymer capacitors designed for high ripple current capabilities (up to 26 A) are available in values up to 1,100 µF, with some manufacturers offering rectangular shapes suitable for stacking into modules.
Multilayer ceramic capacitors (MLCCs) are widely used in power supply filtering and decoupling due to their low ESR and ESL. Continuous improvements in volumetric efficiency have resulted in components like the 1608M (1.6 mm x 0.8 mm) size MLCC with a 1 µF/100 V rating, saving significant volume and surface area compared to previous models.
Recent developments in MLCC packaging technology have enabled bonding without metal frames, maintaining low ESR, ESL, and thermal resistance. Ceramic capacitors with dielectric materials that exhibit minimal capacitance shift with voltage and predictable, linear capacitance changes with temperature are preferred for filtering and decoupling applications.
Conclusion
The need for high processor performance in AI systems imposes specific demands on passive and electromechanical components. These components must be selected with a focus on high-speed data transfer, efficient power delivery, thermal management, reliability, signal integrity, size constraints, and the specific requirements of AI applications, ensuring the electronic system meets the demands of AI workloads effectively and reliably.
Story Credit: Avnet
The post AI to Transform Passive and Interconnect Design appeared first on ELE Times.
Enhancing Analog Input Module Performance in PLCs
To maximize the performance of analog input modules in programmable logic controllers (PLCs) used in factory automation, it is essential to focus on several key design considerations. These modules, which convert real-world signals into digital signals, vary based on voltage and current inputs, channel count, and configuration (single-ended or differential). Below are some important factors for consideration:
- System Accuracy and Reproducibility:
- Precision in analog-to-digital converters (ADCs) and the analog front-end is crucial for accurate and reproducible measurements.
- Component Selection:
- Low-Offset-Drift Amplifiers: Help maintain an adequate error budget.
- Low-Noise Operational Amplifiers (Op Amps): Achieve a higher effective number of bits or noise-free resolution.
- Instrumentation Amplifiers: Enhance precision in measurements.
- Power Consumption and Data Acquisition Speed:
- Balance between power usage and the speed at which data is acquired and processed.
- Process Technology: Choose technology that offers an acceptable speed-to-power ratio.
- Converter Topology:
- Successive Approximation Register (SAR) Converters: Preferred for efficiency without compromising performance.
- Bipolar Technology: Offers high efficiency with a good speed-to-power ratio, but be mindful of input bias current and input impedance.
In systems where a multiplexer allows one measurement at a time, issues such as large differential voltages at the op-amp input can arise, especially when switching between measurements (e.g., pressure and temperature). This can forward-bias input protection diodes, causing leakage currents that affect the amplifier’s settling time and digitization accuracy.
Multiplexer-Friendly Op Amps:
- Employ different protection schemes to avoid the issues caused by internal diodes, improving overall settling time.
When interfacing with field transmitters that have high impedances (greater than 1 MΩ):
- MOSFET Input Op Amps: Suitable for high-impedance applications.
- Junction FET (JFET) Input Amplifiers: Offer very high input impedance but have a narrower common-mode input voltage range.
- Bipolar Op Amps: Provide the best speed-to-power ratio without compromising noise performance but have trade-offs like higher input bias current or lower input impedance.
Super Beta Topology:
This approach in bipolar designs can enhance both DC precision and AC performance, making it versatile for various analog input modules within PLCs.
Diagrams and CalculationsFigure 1: Signal Path Feeding to a High-Resolution Converter – Ensures minimal loss and distortion.
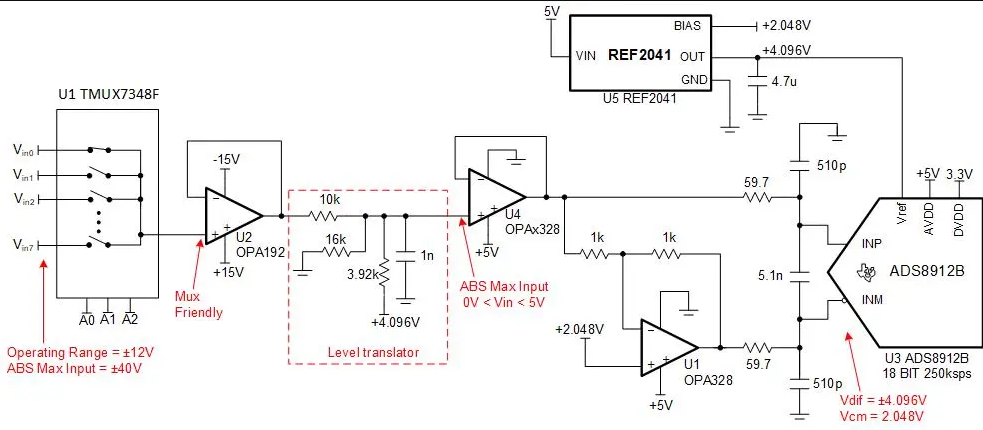
Figure 2: Precision Analog Front-End- Maintains linearity in the converter.
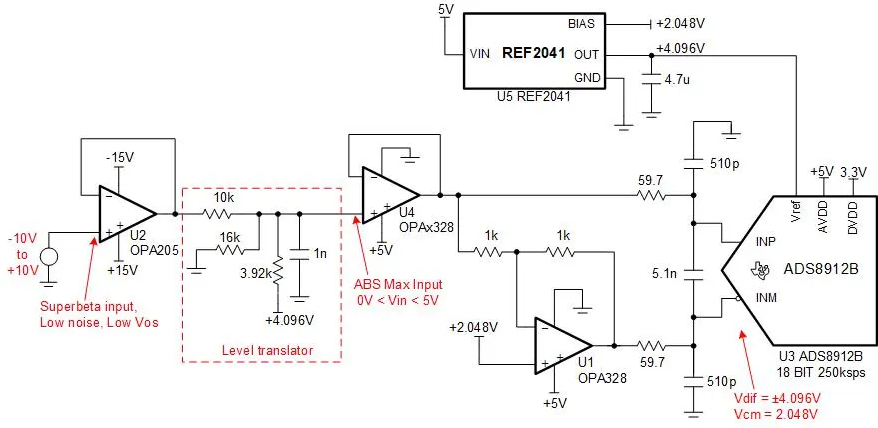
Figure 3: Noise Contributions- Analyzes the impact of amplifiers, voltage reference, and data converter.
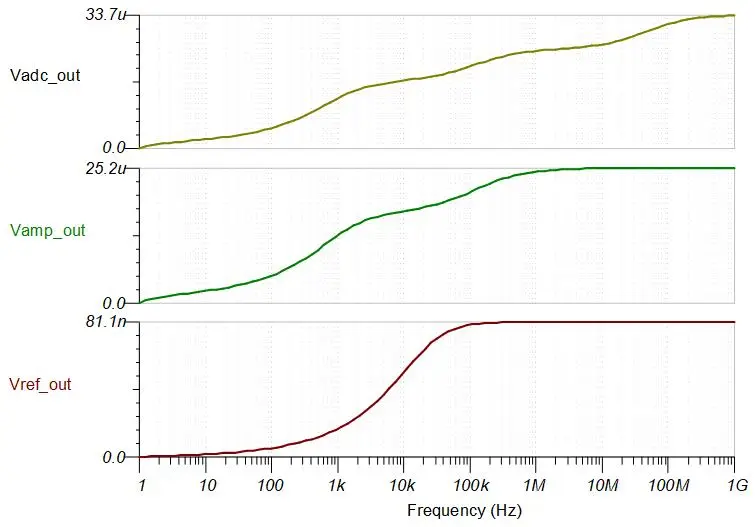
Figure 4: Settling Time: Critical for accurate digitization, calculated as LSB = (4.096 × 2)/2^18, yielding 31.25 mV. Multiplying by 0.5 gives 15.625 mV, representing half an LSB.
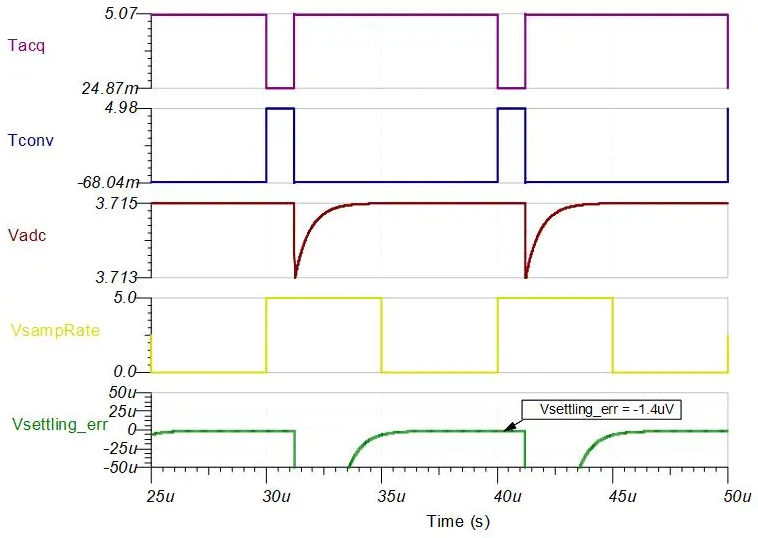
The choice of components will depend on whether the goal is to optimize for settling time or noise performance. A bipolar op amp with integrated overvoltage protection can enhance efficiency without sacrificing settling time. In multiplexed systems, multiplexer-friendly op amps are essential to avoid performance issues.
By carefully selecting and configuring the appropriate components, you can achieve an optimal balance between DC and AC parameters, maximizing the performance of analog input modules in PLCs.
Story Credit: Texas Instruments
The post Enhancing Analog Input Module Performance in PLCs appeared first on ELE Times.
STMicroelectronics Announces Timing for Second Quarter 2024 Earnings Release and Conference Call
STMicroelectronics, a global semiconductor leader serving customers across the spectrum of electronics applications, announced that it will release second-quarter 2024 earnings before the opening of trading on the European Stock Exchanges on July 25, 2024.
The press release will be available immediately after the release on the Company’s website at www.st.com.
STMicroelectronics will conduct a conference call with analysts, investors and reporters to discuss its second quarter 2024 financial results and current business outlook on July 25, 2024 at 9:30 a.m. Central European Time (CET) / 3:30 a.m. U.S. Eastern Time (ET).
A live webcast (listen-only mode) of the conference call will be accessible at ST’s website, https://investors.st.com, and will be available for replay until August 9, 2024.
The post STMicroelectronics Announces Timing for Second Quarter 2024 Earnings Release and Conference Call appeared first on ELE Times.
Microchip Releases Its 2023 Sustainability Report Highlighting Its Environmental Stewardship and Social Impact
One of Microchip’s Guiding Values is “Professional Ethics and Social Responsibility Are Practiced,” which means that the company manages its business and treats its customers, employees, shareholders, investors, suppliers, channel partners, community and government in a manner that exemplifies honesty, ethics and integrity. To demonstrate its commitment to this value and to provide transparency to its employees, customers and all other stakeholders, Microchip Technology has released its 2023 Sustainability Report which details its environmental and social impact programs.
Microchip’s Environmental, Social and Governance (ESG) projects are aligned with its business objectives and are implemented to catalyze positive change throughout its value chain via strategic initiatives, strong collaboration with partners and ambitious goals. The company consistently invests in its global workforce and endeavors to drive economic growth, while preserving natural resources and paving the way for a sustainable tomorrow for future generations.
“Our vision to be the leading supplier of smart, connected and secure embedded control and processing solutions includes a corporate commitment to acting in an ethical and responsible way. This permeates what we do, from day-to-day operations to ethical global supply chains to investing and developing new technologies and products to ensure a more sustainable world,” said Ganesh Moorthy, president and CEO of Microchip. “We have a dedicated Sustainability Megatrend team that is focused on empowering innovation for our customers through a wide range of products and solutions that help reduce power consumption, increase efficiency and reduce waste.”
The company’s 2023 Sustainability Report outlines its ESG efforts and progress across five tenets, referred to as Microchip’s 360° Sustainability Approach. The five tenets include: Our Company, Our Planet, Our Supply Chain, Our Products and Our People.
“To protect our planet, we seek innovative and meaningful ways to improve our environmental impact in four essential areas: greenhouse gas emissions, energy efficiency, water management and waste diversion,” said Ewa Rickey, associate director of Microchip’s ESG program. “Our 2023 Sustainability Report demonstrates our dedication to being a good corporate citizen, not only in the communities where we operate but around the world.”
Highlights of Microchip’s 2023 Sustainability Report- Microchip’s reaffirmation of its ambitious target to achieve a 50% reduction in Scope 1 and 2 Greenhouse Gas (GHG) Emissions by 2030 and to be Net Zero by 2040
- The completed construction of a cutting-edge 3.8 MW floating solar farm at Microchip’s Thailand facility, accompanied by signing a 20-year Power Purchase Agreement (PPA) to source 16% of its electricity needs from this solar farm to affirm the company’s commitment to sustainable energy practices
- The ongoing application of Microchip’s Supply Chain Management Approach, which is used to assess the practices and ethics of supply chain partners to ensure transparency and minimize risk
- An overview of Microchip’s many initiatives aimed at fostering a diverse and inclusive workplace, with a particular focus on employee well-being and professional development
- The company’s community involvement initiatives such as charitable donations and employee volunteer hours, as well as its focus on STEM education and support for the FIRST® Robotics program
- Microchip’s recognition by numerous third-party publications and organizations that included ratings as one of “America’s Most Responsible Companies” by Newsweek and a “World’s Top Female-Friendly Companies” by Forbes/Statista
In line with the company’s Guiding Value, “Continuous Improvement is Essential,” Microchip proactively pursues new avenues to further reduce its environmental impact, enhance its social contributions and strengthen its governance practices. Through its Sustainability Megatrend team, the company prioritizes developing innovative solutions and products that can contribute to sustainability ecosystems such as energy generation, E-Mobility, sustainable homes and cities. To learn more, visit Microchip’s Sustainability Solutions website.
To read Microchip’s 2023 Sustainability Report and to learn more about its ESG initiatives, visit the Corporate Responsibility website.
The post Microchip Releases Its 2023 Sustainability Report Highlighting Its Environmental Stewardship and Social Impact appeared first on ELE Times.
STMicroelectronics reveals ST BrightSense image sensor ecosystem for advanced camera performance everywhere
Enables quicker and smarter designs of compact power-efficient products for factory automation, robotics, AR/VR, and medical applications
STMicroelectronics has introduced a set of plug-and-play hardware kits, evaluation camera modules and software that ease development with its ST BrightSense global-shutter image sensors. The ecosystem lets developers of mass-market industrial and consumer applications ensure superior camera performance by designing-in ST BrightSense image sensors. By sampling all pixels simultaneously, unlike a conventional rolling shutter, global-shutter sensors can capture images of fast-moving objects without distortion and significantly reduce power when coupled to a lighting system.
ST BrightSense CMOS global-shutter sensors implement advanced backside-illuminated pixel technology, manufactured at ST’s own foundry in France, ensuring high image sharpness to capture fine details such as in barcode reading. Their high sensitivity enhances low-light performance and permits fast image capture, enhancing responses such as obstacle avoidance in mobile robots and face recognition in personal electronics. The sensors’ advanced 3D-stacked construction allows an extremely small die area, easing integration anywhere space is limited especially in the final optical module, while enriching the products with advanced on-chip image processing for auto-exposure, correction, and calibration. Their MIPI-CSI-2 interface makes them ideal for embedded vision and edge AI devices.
Historically only offered to qualified customers, ST’s cutting-edge sensor technologies are now available in a wide variety of markets through the ST BrightSense portfolio, highlighting industrial-grade products and 10-year longevity commitment. Widespread access to these sensors, whose qualities are proven with over one billion units shipped on market, now lets developers bring high-performance machine vision to applications that face strict size and power constraints and challenging operating conditions. These include factory automation, scanning, domestic and industrial robots, VR/AR equipment, traffic monitoring, and medical devices.
ST’s new mass-market offering includes evaluation camera modules that integrate image sensor, lens holder, lens, and plug-and-play flex connector to enable instant integration of the image sensors. The modules offer a selection of tiny form factors down to 5mm2, various lens options to suit different application requirements, and a plug-and-play connector that allows easy swapping. A series of hardware kits helps developers integrate the sensors with various desktop and embedded computing platforms. Complementary software tools are available for free download on ST’s website, such as a PC-based GUI and Linux drivers that assist integration with popular processing platforms including STM32MP2 microprocessors.
The ST BrightSense global-shutter family currently comprises the VD55G0, VD55G1, and VD56G3 monochrome sensors with resolution from 0.38Mpixel to 1.5Mpixel, as well as the colour VD66GY with 1.5Mpixel. The sensors, along with their evaluation camera modules, and development boards are in production now. Contact your ST sales office or local distributor contact for pricing options and sample requests.
Webinar on demand (upon free registration) can be accessed here.
For more information, visit www.st.com/brightsense
The post STMicroelectronics reveals ST BrightSense image sensor ecosystem for advanced camera performance everywhere appeared first on ELE Times.
Infineon and Swoboda cooperate to develop high-performance current sensors for electromobility
Infineon Technologies AG and Swoboda jointly develop and market high-performance current sensor modules for automotive applications. The partnership combines the best-in-class current sensor ICs from Infineon with Swoboda’s expertise in the development and industrialization of sensor modules to address the fast-growing market of sensing solutions for hybrid and electric vehicles. The collaborative high-performance current measurement solutions accelerate time-to-market for high-volume applications such as traction inverters and battery management systems, but also for other key automotive applications.
The first of these products, the Swoboda CSM510HP2, features a fully encapsulated current sensor module with significantly reduced footprint without compromising performance. It is based on the Infineon TLE4973 coreless current sensor IC and allows high precision sensing with a total error below 2 percent. The module is specifically designed for seamless integration with the HybridPACK Drive G2, Infineon’s automotive power module for traction inverters in electric vehicles. This eliminates the need for separate external current sensors and thereby enables the most compact traction inverters in the market.
“This new generation of current sensors sets new standards in regards of simplified system integration”, said Klaus Lebherz, Head of Business Development at Swoboda. “Infineon’s latest sensor technology and our injection molding know how turned out to be a perfect match”.
“The market demands high performance, compact and easy to integrate current sensing solutions” said Andrea Monterastelli, Head of Automotive Magnetic Sensors Product Group at Infineon Technologies. “The seamless fit into Infineon`s HybridPack Drive G2 offers the most compact solution for hybrid and electric vehicles, enabled by our best-in-class current sensor ICs and Swoboda’s expertise.”
Infineon and Swoboda are currently developing additional current sensing solutions to serve any kind of power devices. Other target applications include inverter DC link and high voltage battery management among others.
The post Infineon and Swoboda cooperate to develop high-performance current sensors for electromobility appeared first on ELE Times.
Industry-Leading Portfolio of PXI Digital I/O Modules Deliver Enhanced Density, Voltage, and Current Capability
Pickering Interfaces, a leader in modular signal switching and simulation products for electronic test and verification, has introduced four new industrial digital I/O families for PXI- and LXI-based systems. These additions enhance Pickering’s industrial digital input and output modules range, offering higher density, expanded voltage and current ranges, and programmable logic levels in PXI and PXIe form factors. With these new modules, Pickering now holds the industry’s largest and most comprehensive portfolio of PXI and PXIe digital I/O modules.
Digital input and output modules are crucial in automated test systems for operating external devices or interfacing with external logic. Digital inputs test signal voltage levels, while digital outputs can act as a current source, sink, or both. These industrial digital I/O modules are suitable for acquiring signals from digital sensors, and communicating with industrial devices like PLCs, and driving relays, solenoids, and lamps.
“At Pickering Interfaces, we’re committed to providing the largest and most capable catalogue of industrial digital I/O products in the industry,” said Paul Bovingdon, Pickering’s Technical Engineering Manager. “These new modules reflect our commitment to meeting the evolving needs of our customers.”
High-Density Digital Output Modules (Model 40/42-412A)
These high-channel-count output modules expand the existing 40-412 PXI range, adding a PXIe option (model 42-412A). This family offers up to 64 output channels (with 16, 32, 48, or 64 output channel variants), featuring an external maximum output voltage range of 50V and 0.5A low-side or high-side driving capability.
High-Density Digital Input Module with Dual Programmable Thresholds (Model 40/42-414)
The new 40-414 (PXI) and 42-414 (PXIe) digital input modules offer high channel count, high input voltage capability, and dual programmable threshold settings per bank. Independent banks of eight channels allow for multiple voltage level detection across the module. Dual thresholds enable convenient status determination of digital signals by comparing each input voltage to two programmable threshold levels, useful in functional test applications, often replacing the need for a DMM. Available variants include 32, 64, 96, or 128 input channels with maximum threshold voltages of 50V, 100V, 200V, or 300V. These modules effectively determine the state of industrial digital signals in automotive, aerospace, and rail applications, reducing external signal conditioning circuits and minimizing test system complexity and footprint.
Semi-Dynamic Digital I/O Module (Model 40/42-419)
The 40-419 (PXI) and 42-419 (PXIe) digital I/O modules provide 16 to 64 I/O channels with up to a 60V range via external supply or four built-in voltage rail selections (+3.3V, +5V, +12V, or +24V), and up to 300mA current output capability. They also offer I/O direction selection for each port/channel, with each port of eight channels being set as inputs or outputs together, or individual channels within a port being set as input or output. The module provides semi-dynamic 8-bit pattern acquisition and generation using on-board memory.
Relay Driver Module (Model 40/42-411A)
This update to the existing model 40-411 includes PXIe options, offering variants with 16 to 64 low-side outputs, 60V, 1A relay driving capability, and three options for on-board relay voltage supply (plus a mixed voltage option) for low-power applications. The 40-411A (PXI) and 42-411A (PXIe) models are designed to drive external relays from a PXI or PXIe system or Pickering Interfaces’ LXI modular chassis.
Models 40/42-411A, 412A, and 419 feature built-in protection systems, including over-current detection and over-voltage clamp or thermal overload. These modules can drive relay coils without flyback diodes or prevent relay coil current from flowing back into the chassis backplane using an isolation barrier. They offer flexibility with external or internal supply options and high-side or low-side driving capability to meet various load capacity requirements.
The modules’ front panels use a 78-pin D-type connector for models 40/42-411A and 412A or a 160-pin DIN 41612 for models 40/42-414 and 419, supported by a range of connector accessories.
The post Industry-Leading Portfolio of PXI Digital I/O Modules Deliver Enhanced Density, Voltage, and Current Capability appeared first on ELE Times.
The Evolution from Industry 4.0 to Industry 5.0
The manufacturing industry has always been at the forefront of technological innovations. From the advent of the steam engine in the 1700s to the invention of the assembly line by Henry Ford, manufacturing has been one of society’s greatest drivers of change.
Today, the manufacturing industry is once again experiencing a series of unprecedented changes.
The proliferation of robotics, advanced sensors, device connectivity, and advanced analytics has led to modern Industry 4.0 manufacturing. Now, the field is poised for yet another change, with a transition into the world of Industry 5.0.
This blog discusses the differences between Industry 4.0 and Industry 5.0, the challenges facing the evolution from Industry 4.0 to Industry 5.0, and some ways to make the transition as seamless as possible.
Industry 4.0 Versus Industry 5.0At present, most modern factories are classified as “Industry 4.0,” and are commonly referred to as “Smart Factories.”
Industry 4.0 is marked by a significant shift toward a more interconnected and intelligent manufacturing environment, leveraging advancements such as the Internet of Things (IoT), artificial intelligence (AI), cloud computing, and edge computing. This era introduces the capability to gather, analyze, and use vast amounts of data in real time, which enhances decision-making processes, predictive maintenance, and overall operational efficiency. Ultimately, the result is a comprehensive advancement over Industry 3.0 to create more agile, efficient, and responsive manufacturing systems. However, Industry 4.0 remains focused mainly on the manufacturing side of things, answering questions about how to make more products faster, more consistently, of better quality, and at lower cost.
Industry 5.0 expands the technological advancements of Industry 4.0 and augments them by considering human factors. It seeks to redefine roles within manufacturing and beyond—encompassing supply chains and entire operational landscapes—to create a more cohesive, adaptive, human-centric, and sustainable industrial environment.
This paradigm shift moves beyond viewing machines as mere tools for productivity and advocates for a collaborative synergy in which technology enhances human capabilities, creativity, and decision-making processes. The fundamental aim is to achieve a balanced symbiosis between advanced technological systems and the unique insights and values humans bring to the table, ensuring that industrial progress supports both efficiency and the well-being of society at-large. Within this industrial revolution, a significant focus lies on fostering seamless communication and interactions between humans and machines.
Drivers of the EvolutionWhile the differences between Industry 4.0 and Industry 5.0 are clear and defined, understanding the larger societal and geopolitical factors driving this evolution is essential.
One major driver of Industry 5.0 is resilience in the face of global challenges.
Starting in 2020, the COVID-19 pandemic and subsequent disruptions exposed vulnerabilities within global manufacturing networks. In the face of these challenges, Industry 5.0 emphasizes resilience and flexibility, aiming to build systems that can adapt and recover from unforeseen events more efficiently.
In a similar vein, the COVID-19 pandemic heightened consciousness surrounding worker health and safety. Industry 5.0 seeks to leverage the technological advancements of Industry 4.0—namely, sensors and vision systems—to mitigate risks, create safer work environments, and minimize accidents.
Naturally, another significant driver of this evolution is the demand for more innovative, sustainable, and reliable products that can reach the market faster. But how will Industry 5.0 enable these changes?
Industry 5.0 will leverage the integration of digital twins with generative AI, which simplifies the prototyping of new concepts. This integration accelerates the development process and enhances the quality of the final product by allowing for the evaluation of multiple design options in terms of cost, performance, quality, and durability before a product reaches the market. Furthermore, Industry 5.0 has a major focus on sustainability, with products designed to be more recyclable and less polluting. Thus, driven by technological advancements and changing consumer demands, Industry 5.0 holds the promise of delivering innovative, high-quality, and sustainable products more efficiently.
Hurdles for the EvolutionDespite the clear benefits and reasons for moving toward Industry 5.0, the evolution is not without its challenges. Paradoxically, the still ongoing adoption of Industry 4.0 is a major hurdle to adopting Industry 5.0.
Between the advent of Industry 3.0 and the emergence of Industry 4.0, nearly four decades elapsed. Remarkably, in just one decade, we transitioned from Industry 4.0 to the dawn of Industry 5.0, a testament to the rapid pace of technological advancement and its transformative impact on manufacturing and production.
Consequently, now we are witnessing an overlap in which many companies are still in proof-of-concept evaluations of Industry 4.0 while others are leveraging Industry 5.0 already. Currently, while large corporations have made substantial investments in Industry 4.0, the adoption rate among smaller manufacturing sites and processes through full-scale digitalization projects remains relatively low.
Major barriers to adopting of Industry 4.0 technologies include the high capital expenditures needed to purchase new equipment, as well as new skill sets and expertise, along with a lack of trust in modern paradigms such as cloud computing and reliance on data security. Until the industry at large fully embraces Industry 4.0, Industry 5.0 will take some time to reach widespread adoption.
Another challenge of adopting Industry 5.0 is that it represents an inherently different mindset and approach to manufacturing.
Unlike previous evolutions (e.g., Industry 3.0 to Industry 4.0), the Industry 4.0 to Industry 5.0 evolution is not so much a challenge of technological advancement but more so a challenge of mindset advancement. Integrating new digitalization projects requires strong alignment all the way from the chief executive officer to the operator to the manufacturing floor. The organization needs to have a clear vision of what its version of Industry 5.0 will look like and then deploy this idea at all levels. This calls for new processes and ways of operating, including more delegation from the operators to those on the floor.
ConclusionLike the many industrial revolutions in human history, modern manufacturing is currently on the precipice of another remarkable evolution. The transition from Industry 4.0 to Industry 5.0 marks a significant change in the manufacturing world.
Achieving this transition requires an industry-wide mindset shift in how our factories operate, how workers interact with machinery, and how the whole ecosystem can converge. Once this happens, we can enter the era of Industry 5.0, enabling greater sustainability and resilience, increased worker safety, and a more robust supply chain.
The post The Evolution from Industry 4.0 to Industry 5.0 appeared first on ELE Times.