Українською
  In English
ELE Times


Anritsu joins OpenROADM as Test & Measurement instrument vendor
Anritsu Corporation has joined the OpenROADM Multi-Source Agreement (MSA), which defines specifications that facilitate multi-vendor interoperability for optical transmission networks. Anritsu’s participation in these efforts aims to enhance efficiency and flexibility of the optical transmission network. As Test & Measurement instrument vendor, Anritsu’s OpenROADM activities contribute to the openness and efficiency of optical transmission networks by promoting interconnect specifications and interoperability verification.
“Anritsu is thrilled to become the inaugural Test & Measurement instrument vendor in the OpenROADM MSA, comprising service providers and vendors. The OpenROADM MSA Group is committed to the open evolution of network management and vendor interoperability. We eagerly anticipate collaborating on network orchestration, which will enable us to control and monitor the quality of the entire network. Additionally, we are excited about the prospect of introducing test & measurement innovations facilitating network fault detection and causal analysis.” says Tadanori Nishikobara, Marketing Director of the Service Infrastructure Solutions Division at Anritsu Corporation.
Anritsu contributes to OpenROADM activities through test & measurement proposals and support for interoperability verification.
The post Anritsu joins OpenROADM as Test & Measurement instrument vendor appeared first on ELE Times.
Keysight’s FieldFox Introduces Portable Millimeter-wave Analysis with Virginia Diodes Extenders
- Collaboration enables FieldFox handheld analyzers to support up to 170 GHz, offering a rugged and lightweight portable solution for millimeter-wave signal analysis
- Provides in field-testing capabilities for aerospace and defense applications reducing development time
Keysight Technologies, Inc. has expanded the frequency range of its FieldFox handheld signal analyzers, offering up to 170 GHz support for millimeter-wave (mmWave) signal analysis. Through a collaboration with Virginia Diodes Inc. (VDI), Keysight’s A- and B-Series FieldFox handheld analyzers with 18 GHz or higher, can be paired with VDI PSAX frequency extenders to cover sub-THz frequency range.
Field based engineers need precise mmWave measurements to advance modern wireless communications and radar systems. This is critical when it comes to 5G, 6G, aerospace and defense and automotive radar transmission/receiving tests. However, mmWave signals are highly sensitive to obstacles, weather conditions, and interference. Understanding their propagation characteristics through precise measurements helps engineers design more efficient networks and radar systems, improving coverage, and enhancing reliability.
To gain this insight, traditional tools typically include large desktop signal analyzers and generators, which are often very expensive and cumbersome for field measurement use cases. The Keysight FieldFox addresses this issue, enabling mmWave measurements in a lightweight portable solution, when paired with VDI’s PSAX frequency extender modules. In addition, engineers can opt for the FieldFox equipped with the downloadable Option 357 pulse generator, which can be paired with a PSGX module from VDI, to also offer a mmWave signal generation solution up to 170 GHz. This enables users to obtain accurate mmWave measurements in a simple, easy to use and rugged solution.
Key benefits of Keysight’s FieldFox combined with VDI frequency extender modules include:
- Expanded frequency coverage: Expanding the FieldFox’s frequency coverage from as low as 18 GHz, depending on models, up to 170 GHz for either signal analysis or generation.
- Optimized performance at the mmWave range: Supporting in-band signal analysis with selection of spectrum analyzer mode, IQ analyzer mode, or real-time spectrum analyzer (RTSA) mode with extraordinary sensitivity of -155 dBm/Hz typical value.
- Cost efficiency: Compared to traditional mmWave signal analysis and generation solutions, the combination of Keysight FieldFox and VDI frequency extenders reduces costs by half or more.
- Portable and convenient testing: Weighing approximately less than 4 kg in total, the combination of Keysight FieldFox and VDI frequency extenders makes the mmWave field testing much more feasible and convenient for both field and lab environment.
Dr. Thomas W. Crowe, CEO of VDI, said: “VDI manufactures state-of-the-art test and measurement equipment for mmWave and THz applications, including vector network analyzer, spectrum analyzer, and signal generator extension modules. These products enhance the capabilities of high-performance microwave measurement tools by extending them to higher frequencies. Through our collaboration with Keysight, VDI is excited to provide frequency extenders for the FieldFox handheld analyzers, offering customers lightweight solutions for both signal analysis and generation in the mmWave range, with exceptional signal quality and measurement integrity.”
Vince Nguyen, Vice President and General Manager, Aerospace, Defense, and Government Solution Group at Keysight, said: “The aerospace, defense, and commercial sectors lack a portable solution which can provide accurate mmWave measurements. As customers explore innovations they need access to higher frequencies in the radio spectrum, including mmWave. Working with VDI, we’ve developed a solution that is easy to test signal analysis and generation in the field as well as in the laboratory.”
Keysight’s FieldFox combined with VDI extenders will be showcased for the first time at the Keysight booth (Hall A3, Stand 506) at electronica 2024, the world’s leading trade fair and conference for electronics, from November 12 to 15, 2024.
The post Keysight’s FieldFox Introduces Portable Millimeter-wave Analysis with Virginia Diodes Extenders appeared first on ELE Times.
Light + LED Expo India 2024 to shine a spotlight on smart, energy-efficient and human-centric lighting solutions for the diverse needs of India’s architecture, infrastructure and more
India’s premier expo, Light + LED Expo India 2024, is set to showcase advanced products in LED and intelligent lighting solutions from 21st to 23rd November 2024 at Yashobhoomi (IICC), Dwarka, Delhi. With 240+ exhibitors from six countries, the B2B event will present innovative solutions for homes, high rises, architecture, infrastructure, and everything in between.
With LED light emerging as a powerful catalyst in India’s energy efficiency journey, its applications have spread from households and industrial spaces to more complex and customised needs for architecture and interior design, urban infrastructure and smart city projects. Government-backed initiatives like Unnat Jyoti by Affordable LEDs for All and LED Street Lighting National Programme (SLNP), gave a substantial boost to LED adoption in India while saving costs and energy with environmental benefits. Besides this, the growth of the semiconductor ecosystem in India will revolutionise LED manufacturing, boost domestic production, achieve energy efficiency and contribute to positioning India as a global manufacturing hub for semiconductors and diverse lighting products.
Bringing together India’s lighting and LED ecosystem, the 29th edition of Light + LED Expo India will feature 240+ exhibitors and about 1,000+ brands showcasing their products, across a gross area of 14,000 sqm at Yashobhoomi, Delhi, Dwarka. Taking the expo to a whole new level, this year the show will feature 126 new exhibitors and has more participation from lighting automation companies than the previous editions, enhancing the solutions portfolio. Besides India, the expo will present participation from six countries including China, Finland, Germany, Italy, Taiwan and the UAE. Participants include brands like BAG, Calcom, Caterlux, JN Lighting, Kevin Electrochem, Lumens Technologies, Optiks Mechatronics, Power Pallazo, Talenteq, Tinge, Uniglobus, Zylos and many other prestigious names.
Ahead of the show, Shri Piyush Goyal, Hon’ble Minister of Commerce and Industry, Government of India, expressed his thoughts: “The Government of India has undertaken collaborative initiatives to establish a strong LED ecosystem in India. Initiatives like the Unnat Jyoti by Affordable LEDs for All (UJALA) and LED Street Lighting National Programme (SLNP) have significantly bolstered the adoption of energy-efficient lighting solutions across the nation, leading to widespread cost savings, reduced energy consumption and environmental benefits. I am hopeful that this expo and summit will serve as an ideal platform for stakeholders to come together, exchange ideas, showcase advancements and further contribute to the growth and sustainability of the LED industry in India.”
The upcoming event will present dynamic workshops and expert-led sessions, a certification workshop and a conference. The knowledge sessions have been planned in association with the Illuminating Engineering Society (IES), Women in Lighting (WIL), Lighting Designers Association of India (LiDAI) and Electric Component Manufacturers’ Association (ELCOMA). Attendees will explore the innovative applications of lighting technology within India’s cultural landscape, covering topics such as the role of lighting in architecture, the future of entertainment lighting, and advancements in connected lighting systems. The sessions will also address the creative potential of drone light shows, smart lighting solutions, human-centric designs, and India’s emergence as a global lighting hub. Key discussions on ‘circadian lighting’ and the evolving language of lighting design promise attendees’ valuable insights into the industry’s latest trends and technologies.
Mr Parag Bhatnagar, President, of the Electric Lamp and Component Manufacturers Association of India (ELCOMA) commented: “In the last few years there were some disruptions because of the technology changes, however, there are many opportunities as India is growing. You look at any segment whether it is infrastructure or modern office space which is growing by 30% or even the recent PLI scheme of the government – the CAPEX cycle is triggered and there is growth in the industry. There is an opportunity to upgrade the industry. I think Light + LED Expo India is a very prominent platform where the entire lighting fraternity will come together and there will be knowledge sharing around government policies, standards and innovation and especially, the new initiatives around lighting design. I invite the entire lighting fraternity and all ELCOMA members to Light + LED Expo India taking place from 21 – 23 November 2024 at Yashobhoomi (IICC), Dwarka, Delhi.”
What makes the expo more relevant is the fact that the Indian LED lighting industry in 2023 stood at USD 4.2 billion which is expected to reach USD 23.2 Billion by 2032, growing at a rate of 20.91% during 2023-2032, according to a report from Research and Markets. Mr Raj Manek, Executive Director and Board Member, Messe Frankfurt Asia Holdings Ltd, shared: “According to leading industry research companies, the LED lighting industry is expected to grow about five-fold by 2032. At this juncture, the Light + LED Expo India will exhibit an array of products and solutions before the industry, with new launches that will take place at the show. Entering the 29th edition, the expo has received a great response from the participating companies ready to display an advanced showcase that will aid in addressing the evolving needs of the Indian landscape. I feel proud that the show highlights the growth of India’s lighting and LED industry with an emphasis on sustainability in the segment.”
The exhibition has garnered strong support from India’s leading industry associations and government bodies such as Ministry of Electronic & Information Technology (MeitY), Ministry of Commerce & Industry, Energy Efficiency Services Limited (EESL) Ministry of Power, LiDAI (Lighting Designers Association of India), CREDAI-MCHI, Council of Architecture (CoA), Luminaire Accessories Components Manufacturers Association (LACMA), Indian Building Congress (IBC), Solar Energy Society of India (SESI), Bombay Suburban Electric Supply (BSES), The Calcutta Electric Traders Association and the Secunderabad Electric Traders Association.
The show is organised by Messe Frankfurt Trade Fairs India Pvt Ltd in association with ELCOMA. Light + LED Expo India is part of Messe Frankfurt’s Light + Building Technology fair portfolio, which is headlined by the biennial Light + Building event in Frankfurt, Germany.
The post Light + LED Expo India 2024 to shine a spotlight on smart, energy-efficient and human-centric lighting solutions for the diverse needs of India’s architecture, infrastructure and more appeared first on ELE Times.
STMicroelectronics’ innovative biosensing technology enables next-generation wearables for individual healthcare and fitness
Highly integrated biosensor device combines input channel for cardio and neurological sensing with motion tracking and embedded AI core
Demonstration to take place at Electronica 2024, Munich, November 12-15
STMicroelectronics, a global semiconductor leader serving customers across the spectrum of electronics applications, has introduced a new bio-sensing chip for the next generations of healthcare wearables like smart watches, sports bands, connected rings, or smart glasses. The ST1VAFE3BX chip combines a high-accuracy biopotential input with ST’s proven inertial sensing and AI core, which performs activity detection in the chip to ensure faster performance with lower power consumption.
“Wearable electronics is the critical enabling technology for the upsurge in individual health awareness and fitness. Today, everyone can have heart-rate monitoring, activity tracking, and geographical location on their wrist,” said Simone Ferri, APMS Group VP, MEMS Sub-Group General Manager at STMicroelectronics. “Our latest biosensor chip now raises the game in wearables, delivering motion and body-signal sensing in an ultra-compact form-factor with frugal power budget.”
Analysts at Yole Development see opportunities for wearable monitors transcending the general wellness market, including consumer healthcare devices that are approved by health organizations and available over the counter. By creating a complete precision sensor input in silicon, ST’s chip-design experts are facilitating innovation in all segments, with advanced capabilities such as heart-rate variability, cognitive function, and mental state.
The ST1VAFE3BX provides opportunities to extend wearable applications beyond the wrist to other locations on the body, such as intelligent patches for lifestyle or medical monitoring purposes. ST customers BM Innovations GmbH (BMI) and Pison are working at the frontiers in this sector and have quickly adopted the new sensor to drive new-product development.
BMI is an electronic design contracting company experienced in wireless sensing and with an extensive portfolio of projects including several leading-edge heart rate and performance monitoring systems. “ST’s new biosensor has enabled us to develop the next generation of precise athlete performance monitoring systems including ECG analysis in a chest band or a small patch,” said Richard Mayerhofer, Managing Director BM innovations GmbH. “Combining the analog signal from the vAFE with motion data from the acceleration sensor within a compact single package facilitates precise and context-aware data analysis. And with additional support for our AI algorithms directly on the sensor, this is exactly what we have been looking for.”
David Cipoletta, CTO of Pison, a developer focusing on advanced technologies to enhance health and human potential, added, “ST’s new biosensor stands out as a great solution for smartwatch gesture recognition, cognitive performance, and neurological health. Leveraging this advancement, we have significantly enhanced the functionality and user experience of our wearable devices.”
The ST1VAFE3BX is in production now in a 2mm x 2mm 12-lead LGA package and available from the eSTore (free samples available) and distributors from $1.50 for orders of 1000 units.
Visitors to Electronica 2024, the major industry trade event happening in Munich November 12-15, can see the ST1VAFE3BX in a sensing technologies demonstration at the ST booth, Hall C3 101. More information is available online at www.st.com/biosensors
Further technical information
The analog front-end circuits for biopotential sensors are difficult to design and subject to unpredictable effects such as skin preparation and the position of electrodes attached to the body. The ST1VAFE3BX provides a complete vertical analog front end (vAFE) that simplifies the detection of different types of vital signs that can indicate physical or emotional state.
Manufacturers of wellness and healthcare devices can thus extend their product ranges to include functionality such as electrocardiography (ECG), electroencephalography (EEG), seismocardiography (SCG), and electroneurography (ENG). This can drive the emergence of new devices that are affordable, easy to use, and reliably indicate health status or physiological responses to events such as stress or excitement. The future could contain a greater diversity of wearable devices that can contribute towards enhanced healthcare, fitness, and self-awareness.
Bringing this precision front end on-chip, the ST1VAFE3BX is building on ST’s established competencies in MEMS (microelectromechanical systems) devices by integrating an accelerometer for inertial sensing. The accelerometer provides information about the wearer’s movement, which is synchronized with the biopotential sensing to help the application infer any link between measured signals and physical activity.
The ST1VAFE3BX also integrates ST’s machine-learning core (MLC) and finite state machine (FSM) that enable product designers to implement simple decision trees for neural processing on the chip. These AI skills let the sensor handle functions such as activity detection autonomously, offloading the main host CPU to accelerate system responses and minimize power consumption. In this way, ST’s sensors let smart devices provide more sophisticated functions and operate for longer between battery charging, enhancing usability. ST also provides software tools like MEMS Studio in the ST Edge AI Suite dedicated to helping designers unleash the maximum performance from the ST1VAFE3BX, including tools for configuring decision trees in the MLC.
The ST1VAFE3BX’s bio-detection signal channel comprises the vAFE with programmable gain and 12-bit ADC resolution. The maximum output data rate of 3200Hz is suitable for a wide variety of biopotential measurements to quantify heart, brain, and muscular activity.
The device is powered from a supply voltage in the range 1.62V to 3.6V and has typical operating current of just 50µA, which can be cut to just 2.2µA in power-saving mode.
The integrated low-noise accelerometer has programmable full-scale range from ±2g to ±16g.
In addition to the machine-learning core and programmable finite state machine, which can provide functionality such as activity detection, the ST1VAFE3BX implements advanced pedometer, step detector, and step counting functions.
The post STMicroelectronics’ innovative biosensing technology enables next-generation wearables for individual healthcare and fitness appeared first on ELE Times.
Registration is Now Open for Microchip’s 17th Annual India MASTERs Conference
Taking place from December 10–13, MASTERs offers over 50 technical sessions for embedded control engineers
Microchip Technology has announced that registration is now open for its signature MASTERs Conference, the premier technical training event for embedded control engineers. The 17th Annual India MASTERs Conference returns to an in-person format and will take place at the Sheraton Grand Bangalore Hotel at Brigade Gateway from December 10–13, 2024.
Commonplace in the semiconductor industry is the use of acronyms and “MASTERs” is no exception. It stands for Microchip Annual Strategic Technical Exchange and Review. The conference strives to deliver advanced technical learning sessions that are taught by application and design engineers to foster a synergistic engineer-to-engineer experience.
The sessions at MASTERs are curated for engineers at all levels of experience and specialties and cover an array of embedded control topics including analog, functional safety, IoT, clock and timing, FPGA solutions and much more. The conference will feature 51 technical sessions of which 22 are hands-on workshops as well as technology showcase sessions for attendees to explore Microchip’s development tools. A highlight of this year’s MASTERs Conference is the networking dinner on December 11, which will feature a keynote by Joe Krawczyk, Microchip’s senior corporate vice president of global sales.
“We are delighted for the long-awaited return of MASTERs and look forward to reconnecting with existing clients and meeting new attendees,” said Edward Han, Microchip’s vice president of Asia Pacific sales. “This year marks the 17th annual India conference and we are proud of the legacy we have built over the years. Microchip strives to inspire and shape the engineers of tomorrow and we hope attendees will leave the conference with a rich and rewarding learning experience.”
Throughout the MASTERs Conference, networking sessions will be available with Microchip engineers at the Ask the Experts booths. These networking sessions provide attendees with the opportunity to meet with Microchip experts to learn about available tools and are given mini lessons on how to use them to fast-track the development of their applications.
MASTERs Registration and Pricing Information
Registration pricing is all inclusive at Rs. 12,000 and includes entry to the conference courses, meals and access to all class materials. Deadline to register is November 26, 2024.
For more information and to register, visit the conference web page.
The post Registration is Now Open for Microchip’s 17th Annual India MASTERs Conference appeared first on ELE Times.
ROHM’s New 1200V IGBTs Achieve Industry-Leading Low Loss Characteristics with High Short-Circuit Tolerance
Contributes to higher efficiency in automotive electric compressors and inverters for industrial equipment
ROHM has developed automotive-grade AEC-Q101 qualified 4th Generation 1200V IGBTs that combine class-leading* low loss characteristics with high short-circuit resistance. This makes the devices ideal for vehicle electric compressors and HV heaters as well as industrial inverters. The current lineup includes four models – RGA80TRX2HR / RGA80TRX2EHR / RGA80TSX2HR / RGA80TSX2EHR – in two discrete package types (TO-247-4L and TO-247N), along with 11 bare chip variants – SG84xxWN – with plans to further expand the lineup in the future.
The increasing use of higher voltages in automotive systems and industrial equipment has led to a growing demand for power devices capable of handling high voltages in applications such as vehicle electric compressors, HV heaters, and inverters for industrial equipment. At the same time, there is a strong push for high efficiency power devices to improve energy conservation, simplified cooling mechanisms, and smaller form factors for a decarbonized society. Automotive electrical components must also comply with automotive reliability standards, while power devices for inverter and heater circuits are required to provide current interruption capabilities during short circuits, necessitating high short-circuit tolerance.
In response, ROHM redesigned the device structure and adopted an appropriate package to develop new 4th Generation IGBTs suitable for high voltage by delivering industry-low loss characteristics with superior short-circuit tolerance. These devices achieve an industry-leading* short-circuit withstand time of 10µs (Tj=25°C) together with low switching and conduction losses while maintaining a high withstand voltage of 1200V and meeting automotive standards by reviewing the device structure, including the peripheral design. At the same time, the new TO-247-4L package products, which feature 4 terminals, can accommodate an effective voltage of 1100V in a ‘Pollution Degree 2 environment’ by ensuring adequate creepage distance between pins. This enables support for higher voltage applications than conventional products.
Implementing creepage distance measures on the device side alleviates the design burden for manufacturers. On top, the TO-247-4L package achieves high-speed switching by including a Kelvin emitter terminal, resulting in even lower losses. In fact, when comparing the efficiency of the new TO-247-4L packages with conventional and standard products in a 3-phase inverter, loss is reduced by about 24% compared to standard products and by 35% over conventional products – contributing to higher efficiency in drive applications.
ROHM will continue to expand its lineup of high-performance IGBTs that contribute to greater miniaturization and high efficiency drive in automotive and industrial equipment applications.
Terminology
AEC-Q101 Automotive Reliability Standard
AEC stands for Automotive Electronics Council, a reliability standard for automotive electronic components established by major automotive manufacturers and US electronic component makers. AEC-Q101 is a standard that specifically applies to discrete semiconductor products (i.e. transistors, diodes).
Short-Circuit Tolerance
The time that a power device can withstand a short-circuit without being destroyed.
IGBT (Insulated Gate Bipolar Transistor)
A power transistor that combines the high-speed switching characteristics of a MOSFET with the low conduction loss of a bipolar transistor.
Creepage Distance
The shortest distance along the surface of an insulator between two conductors. In semiconductor design, insulation measures with such creepage and space distances must be taken to prevent electric shocks, leakage currents, and short-circuits in semiconductor products.
Pollution Degree 2 Environment
Pollution Degree 2 corresponds to typical environments such as homes and offices where only dry, non-conductive contaminants are present. Pollution Degree is the grade of the environment that influences the determination of the spatial and creepage distances of components, classified from 1 to 4 according to the presence, amount, and condition of pollutants.
Kelvin Emitter Terminal
An emitter terminal dedicated to voltage measurement. By separating this from the emitter through which current flows, the effects of voltage drop during current flow can be minimized, allowing for fast, stable switching.
The post ROHM’s New 1200V IGBTs Achieve Industry-Leading Low Loss Characteristics with High Short-Circuit Tolerance appeared first on ELE Times.
Infineon and Stellantis Team Up to Advance Innovation in Power Conversion and Distribution for Next Generation of Vehicle Architectures
- Collaboration aims to significantly enhance cost, energy efficiency, driver experience and vehicle range
- Companies signed supply and capacity reservations for PROFET power switches and silicon carbide (SiC) CoolSiC semiconductors
- Infineon’s scalable production capacity is ready to meet the market demand for automotive semiconductor solutions
Stellantis N.V. and Infineon Technologies AG announced that they will work jointly on the power architecture for Stellantis’ electric vehicles to support Stellantis’ ambition of offering clean, safe and affordable mobility to all.
To support this, the companies have signed major supply and capacity agreements that will serve as the foundation for the planned collaboration to develop the next generation of power architecture, including:
- Infineon’s PROFET smart power switches, which will replace traditional fuses, reduce wiring and enable Stellantis to become one of the first automakers to implement intelligent power network management.
- Silicon carbide (SiC) semiconductors, which will support Stellantis in its efforts to standardize its power modules, improve the performance and efficiency of EVs while also reducing costs.
- AURIX microcontrollers, which target the first generation of the STLA Brain zonal architecture.
Stellantis and Infineon are also in the process of extending their cooperation with the implementation of a Joint Power Lab to define the next-generation scalable and intelligent power architecture enabling Stellantis’ software-defined vehicle.
“As outlined in our strategic plan, Dare Forward 2030, we are securing the supply of crucial semiconductor solutions required to continue our transition to an electrified future leveraging innovative E/E architectures for our next-generation platforms,” said Maxime Picat, Stellantis Chief Purchasing and Supplier Quality Officer.
“Infineon is now entering a collaboration and innovation partnership with Stellantis,” said Peter Schiefer, President of Infineon’s Automotive Division. “As the world’s leading automotive semiconductor vendor, we bring our product-to-system expertise and dependable electronics to the table. Our semiconductors drive the decarbonization and digitalization of mobility. They increase the efficiency of cars and enable software-defined architectures that will significantly improve the user experience.”
With the world`s most cost-competitive SiC fab in Kulim, Malaysia, the upcoming 300-millimeter ”Smart Power Fab” in Dresden, Germany, and the joint venture with TSMC and partners (ESMC) as well as accompanying supply agreements with foundry partners, Infineon is ready to fully meet market demand for automotive semiconductor solutions. According to the market research company TechInsights, Infineon is the global number one supplier of automotive microcontrollers with a market share of about 29 percent of the global automotive microcontroller market.
The post Infineon and Stellantis Team Up to Advance Innovation in Power Conversion and Distribution for Next Generation of Vehicle Architectures appeared first on ELE Times.
STMicroelectronics and ENGIE sign long-term agreement for the supply of electricity produced from renewable sources in Malaysia
- 21-year contract for energy produced by a new solar farm in Malaysia, where ST operates a large-volume test & assembly site.
- PPA will support the 2027 carbon neutrality and 100% renewable energy sourcing targets of ST.
STMicroelectronics, a global semiconductor leader serving customers across the spectrum of electronics applications, announced a 21-year Power Purchase Agreement (PPA) with BKH Solar Sdn Bhd, an entity jointly established by ENGIE Renewable SEA Pte Ltd (ENGIE), a renowned global leader in low-carbon energy and services, and Conextone Energy Sdn Bhd, a rapidly emerging solar energy developer in Malaysia. The agreement will facilitate the supply of approximately 50 GWh of renewable energy annually from a new solar farm in Bukit Kayu Hitam, Kedah, Malaysia. This long-term agreement is undertaken under the Corporate Green Power Program introduced by the Malaysian Single Buyer in 2023.
Geoff West, EVP and Chief Procurement Officer, STMicroelectronics, commented: “This long-term power purchasing agreement in Malaysia, ST’s first one in Asia, marks yet another important step towards ST’s goal of becoming carbon neutral in its operations (Scope 1 and 2 emissions, and partially scope 3) by 2027, including the sourcing of 100% renewable energy by 2027. PPAs will play a major role in our transition. Starting in 2025, this PPA with ENGIE will provide a significant level of renewable energy for ST’s operations in our high-volume test and assembly site in Muar, Johor, Malaysia.”
Amit Jain, Managing Director India & Southeast Asia, ENGIE, commented: “ENGIE is delighted to join forces with STMicroelectronics in the global transition towards sustainable energy solutions. By supplying approximately 50 GWh of renewable energy annually from our new solar PV 30 MW project in Malaysia, we are proudly contributing to ST’s transition towards 100% renewable sourcing. This partnership with ST demonstrates our commitment to providing green, clean and reliable energy to our clients.”
ST’s high-volume test and assembly site located in Muar, Johor, has over 4,700 employees supporting multiple technologies and products, including high reliability applications for automotive customers. ST also operates support functions out of Penang and Kuala Lumpur.
The post STMicroelectronics and ENGIE sign long-term agreement for the supply of electricity produced from renewable sources in Malaysia appeared first on ELE Times.
Renesas Brings the High Performance of Arm Cortex-M85 Processor to Cost-Sensitive Applications with New RA8 Entry-Line MCU Groups
The post Renesas Brings the High Performance of Arm Cortex-M85 Processor to Cost-Sensitive Applications with New RA8 Entry-Line MCU Groups appeared first on ELE Times.
New high-performance microcontroller: Infineon launches AURIX TC4Dx
Infineon Technologies AG has announced the launch of the AURIX TC4Dx microcontroller (MCU), the first member of the latest AURIX TC4x family. Based on 28nm technology, the AURIX TC4Dx offers increased performance and high-speed connectivity. It combines power and performance enhancements with the latest trends in virtualization, Artificial Intelligence, functional safety, cybersecurity, and networking functions paving the way for new Electric/Electronical (E/E) architectures as well as the next generation of software-defined vehicles. MCUs like the AURIX TC4Dx are crucial to control and monitor a wide variety of systems in the automobile such as vehicle motion control, Advanced Driver Assistance Systems (ADAS) and chassis.
“Microcontrollers like our new AURIX TC4Dx are the backbone of software-defined vehicles. They are essential to further improve vehicle performance, safety and comfort.” said Thomas Boehm, Senior Vice President for Microcontrollers at Infineon. “The AURIX TC4Dx will contribute to secured processing performance and efficiency, and our customers will benefit from faster time-to-market and lower total system cost.”
The AURIX TC4Dx features an advanced multi-core architecture with the new 500MHz TriCore with six cores, all with lock-steps for highest functional safety performance. With its Parallel Processing Unit (PPU), the MCU provides an innovative platform for developing embedded AI-based use cases such as motor control, battery management systems or vehicle motion control. The MCU is supported by a strong software ecosystem and includes networking accelerators to boost Ethernet and CAN communication, as well as the latest interfaces such as 5 Gbit/s Ethernet, PCIe, 10Base-T1S and CAN-XL. This increased networking throughput and connectivity gives customers the performance and flexibility needed to implement E/E architectures. Its holistic approach to functional safety meets the highest functional safety requirements according to ISO26262 ASIL-D. The AURIX TC4Dx also fulfills latest cyber security standards according to ISO/SAE21434 including post-quantum cryptography support.
Infineon at electronica 2024
At this year’s electronica in Munich, Infineon presents innovative solutions that are helping to shape an all-electric society. Visitors can explore sustainable technologies that are transforming the mobility and automotive landscape, enabling sustainable buildings and smarter living, and promoting the growth of artificial intelligence with minimal environmental impact. The company will present intelligent and energy-efficient solutions for tomorrow’s connected world from November 12 to 15 in Hall C3, Booth 502 under the motto “Driving Decarbonization and Digitalization.
The post New high-performance microcontroller: Infineon launches AURIX TC4Dx appeared first on ELE Times.
ST Foundation: bridging the digital divide with the Digital Unify Program
At STMicroelectronics, we are committed to positively impacting expertise, and delivering comprehensive educational programs globally through the ST Foundation. The mission is to develop, coordinate, and sponsor projects that use modern sciences and technology to promote human progress.
One of the flagship initiatives is the Digital Unify Program, an extensive educational effort active across Europe, Asia, and Africa. Since its launch in 2003, this program has trained over one million individuals across 29 countries, adapting the methods to meet the needs of various groups, including children, adults, individuals with disabilities, and the elderly.
The impact of the Digital Unify Program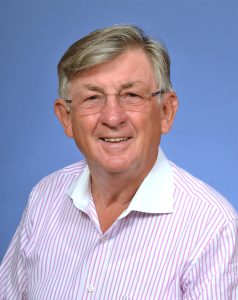
The Digital Unify Program (DU) sets up computer training centers (DU Labs) in collaboration with local partners such as schools, NGOs, local administrations, and government agencies. Currently, the program offers four free courses:
- Informatics and Computer Basics (ICB): a 20-hour computer literacy course focusing on essential skills like searching topics online, sending emails, and using word processors and spreadsheets. Shereen’s story is just one of the many examples of empowerment through education.
- Tablet for Kids (T4K): a course for children aged 9 to 13, aimed at providing an intuitive understanding of ICTs for problem-solving and personal development. Read more about what we are doing to help kids in Murshidabad, one of the most underdeveloped areas in West Bengal (India).
- Introduction to Computer Basics for Visually Impaired People (ICB4VI): created in collaboration with the University of Milan, this course makes digital skills accessible to visually impaired learners. It offers over 50 hours of training on key topics such as keyboard navigation, Microsoft Word document creation, Excel data management, email handling, and Internet navigation.
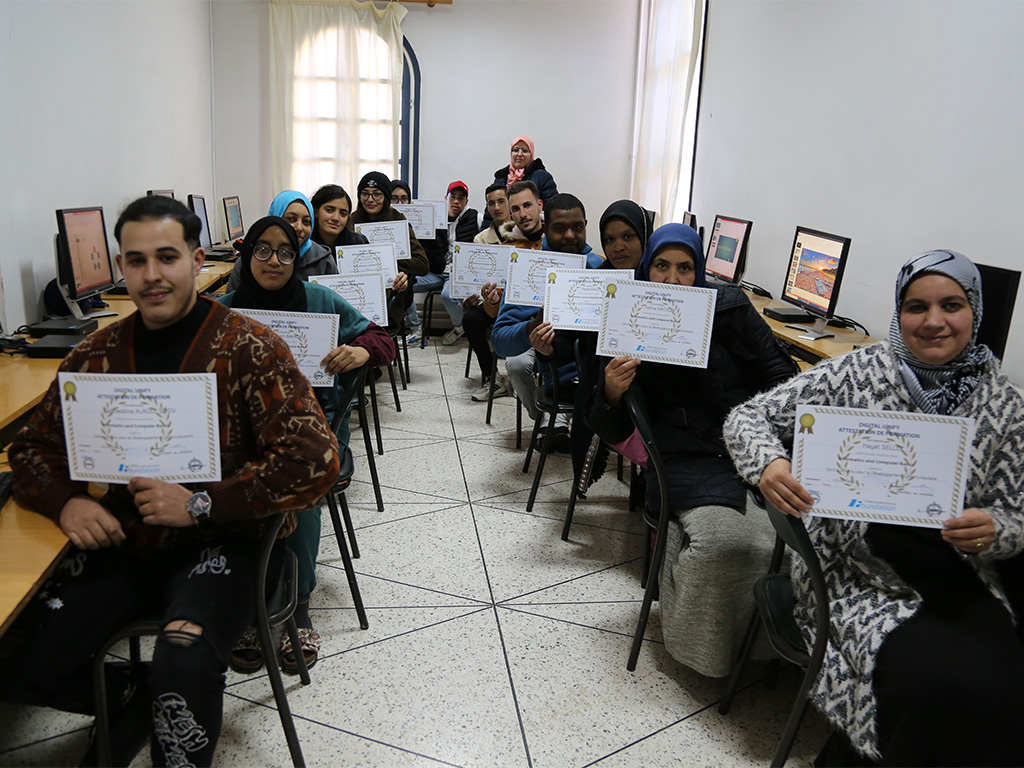
- Tablet for Seniors Course (T4S): This course has a standard duration of 20 hours, during which the beneficiaries have the chance to learn basic skills like how to use the Internet, email accounts, take pictures, and more advanced tasks that can help them lead a more active and e-connected life. Read the story of Remedios in the Philippines to know more about how this course can change people’s lives.
In addition to these fundamental courses, the program remains attentive to the evolving needs of the communities it serves. The ST Foundation is always ready to develop new courses and adapt our offerings to ensure everyone has access to the digital tools they need to meet future challenges.
Creating a better future with DU local communitiesThe Local Communities (LC) project is a key initiative of the ST Foundation. It supports the creation of DU Labs and encourages volunteering among ST employees. The goal is to provide every citizen with the knowledge to overcome social, economic, and technological inequalities, achieving human progress and a dignified life for everyone. If you want to know more about how to connect with the ST Foundation to benefit your local community, click here.
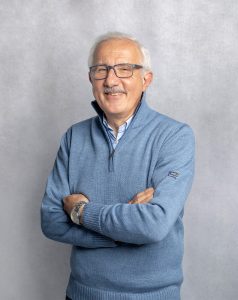
The ST Foundation continues to expand its reach in countries where there is a significant need to overcome social, economic, and technological inequalities.
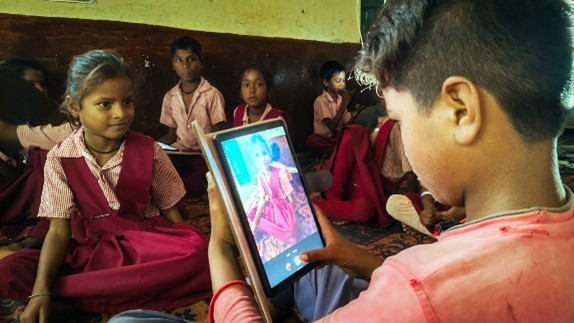
In 2023, the Foundation signed a strategic collaboration agreement with the International Telecommunication Union (ITU) – a specialized agency of the United Nations responsible for issues related to information and communication technologies (ICTs) – strengthening the commitment to reducing the digital divide. This collaboration focuses on empowering marginalized communities, particularly women and youth, through digital skills development. This partnership with ITU aligns with the mission to foster digital inclusion and promote sustainable development across various regions.
In Senegal, the Foundation launched training programs for young girls and visually impaired individuals, ensuring more inclusive access to technology and education. Additionally, they conducted a Training of Trainers (ToT) program in Ghana to equip the first teachers in the country to deliver our ICB4VI course, enhancing accessibility for visually impaired learners.
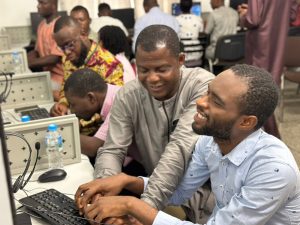
In India, the efforts included expanding the T4S senior citizen program, empowering women with digital literacy, and introducing the Digital Unify program in prisons, aiding rehabilitation and reintegration. The summer camps in Morocco reached 9,502 children across 29 locations, providing quality education and fostering unity among underprivileged children.
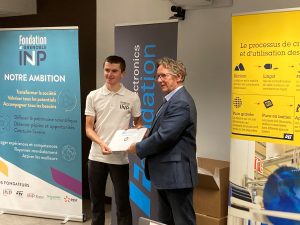
In France, the Foundation donated nearly 1,000 computers and formed partnerships with major entities.
In Italy, they successfully promoted coding in education with a new initiative together with ACRA, encouraged digital careers, and trained volunteers to engage with students. The initiative reached schools, trained teachers, and involved volunteers, focusing on inclusion and reducing inequality.
The collaboration with Vittascience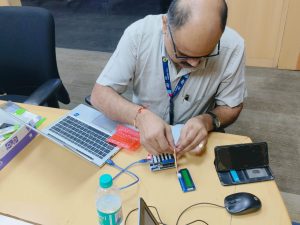
The Foundation has made significant additional strides in 2023, focusing on reducing digital education disparity and enhancing program efficacy. Key projects include developing a new computational thinking and coding course using resources from Vittascience, an educational platform offering innovative teaching tools. The course aims to reinforce existing programs and pay special attention to digital education for older people
Shireen’s and Barthelemy’s journeys and the many active projects in 14 countries are just some of many recent inspiring stories highlighting the importance of providing educational opportunities to less privileged communities. These stories show how access to modern sciences and technology can promote human progress and
The post ST Foundation: bridging the digital divide with the Digital Unify Program appeared first on ELE Times.
Vishay Intertechnology High Energy Inrush Current Limiting PTC Thermistors Increase Performance in Active Charge and Discharge Circuits
Reducing Component Counts, Self-Protecting Devices Combine Energy Handling to 340 J With R25 Values to 1.5 kΩ and High Voltages to 1200 VDC
Vishay Intertechnology, Inc. has introduced a new series of inrush current limiting positive temperature coefficient (PTC) thermistors. Designed to increase performance in active charge and discharge circuits for automotive and industrial applications, Vishay BCcomponents PTCEL High Energy series devices combine maximum energy handling to 340 J — five times higher than competing devices at high ambient temperatures — with a wide range of resistance at 25 °C (R25) values and high voltage capabilities.
Featuring R25 values from 150 Ω to 1.5 k Ω, the thermistors released today enable increased efficiency and high maximum voltages of 1200 VDC. The devices offer high energy handling capabilities at higher ambient temperatures — 180 J at 85 °C and 130 J at 105 °C — allowing designers to save space and lower costs by utilizing fewer components in their circuits. With their high switching temperature and operation to +105 °C, the PTCEL High Energy series offers a heat capacity to 2.6 J/K for all resistance values.
AEC-Q200 qualified and self-protecting — with no risk of over-heating — the thermistors provide current limitation and overload protection in AC/DC and DC/DC converters; DC-Link, battery management, and emergency discharge circuits; on-board chargers; home energy storage systems; heat pumps; motor drives; and welding equipment. For these applications, the devices withstand > 100 000 inrush power cycles and are highly resilient against non-switching peak power up to 25 kW.
The PTCEL High Energy series barium titanate thermistors consist of a ceramic pellet soldered between two tinned CCS wires and coated with a UL 94 V-0 compliant high temperature silicone lacquer. The devices are available in tape on reel packaging and can be automatically handled by pick and place equipment for lower placement costs. The thermistors offer a standard leadwire pitch of 10 mm, with leadwire pitches of 5.0 mm and 7.5 mm also available. SPICE and 3D models for the RoHS-compliant devices are available.
Device Specification Table:
Part number |
PTCEL67R |
R25 (W) |
150 to 1500 |
R25 tolerance (%) |
30 |
Max. AC voltage (VRMS) |
460 to 800 |
Max. DC voltage (VDC) |
650 to 1200 |
Maximum energy (J) @ 25 °C |
340 |
Heat capacity (J/K) |
2.6 |
Lead pitch (mm) |
5.0, 7.5, 10.0 |
The post Vishay Intertechnology High Energy Inrush Current Limiting PTC Thermistors Increase Performance in Active Charge and Discharge Circuits appeared first on ELE Times.
STPOWER Studio: 3 new topologies for accurate electro-thermal simulation on STPOWER devices
STPOWER Studio 4.0 just became available and now supports three new topologies (1-phase full bridge, 1-phase half-bridge, and 3-phase 3-level T-NPC) to cover significantly more applications. Previously, the online simulation tool only offered a 3-phase 2-level topology for motor drivers and photovoltaic inverters, which are some of the most popular use cases. Thanks to the robustness of the underlying architecture that ST recently updated and brought to the web, we are now able to build on top of the existing platform to make STPOWER Studio more versatile and assist more engineers in designing a more comprehensive range of power stages.
What is STPOWER Studio? A unique simulator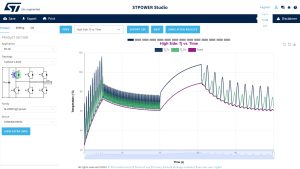
STPOWER Studio, a component of eDesignSuite, specializes in thermo-electrical simulations. As eDesignSuite transitioned to HTML5 to enhance its user interface, STPOWER Studio benefited from the same underlying architecture, enabling more powerful simulations. It stands out in the industry as one of the few simulators capable of adjusting power losses according to the junction temperature at the moment of the simulation, providing a more accurate representation of real-world usage. Some competitors traditionally use a fixed value for the junction temperature, leading to over- or underestimated losses. Thanks to our dynamic junction temperature, users get more accurate results.
STPOWER Studio can simulate up to hundreds of seconds per step with Steady State off, which gives engineers enough time to see how their power stage would ramp up and stabilize. They can also run simulations with or without heat sinks, which will help them anticipate form factor and heat dissipation requirements. Users simply select the ST family of devices (ACEPACK or SLLIMM) and the component they will use in their design. Under Setting, designers can tweak the gate resistor values and some thermal properties. Finally, under I/O, users can adjust their mission profile by defining various steps with values such as the output power or the current level, among other things.
A design assistantLet’s take the example of an engineer designing a large motor driver for industrial applications, an inverter for a photovoltaic converter, or an HVAC system. In our example, the motor would use a DC Link voltage of 650 V and an RMS Phase Current of 10 A. For a quick simulation, users can use Steady State ON to analyze performances after reaching a thermal steady state. Then, by choosing Steady State OFF, users can set the duration of the simulation step for a more detailed analysis. Obviously, it will require more computing power on the server and take longer to generate. However, ST reduced rendering times by a factor of 10 over the last releases of STPOWER Studio.
What’s new in STPOWER Studio 4.0? 1-phase full bridge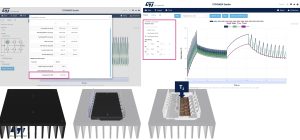
The new version of STPOWER Studio features three new topologies. The 1-phase full bridge will fit single-phase photovoltaic converters or uninterruptible power supplies. As more residential homes and buildings increasingly rely on renewable energy, the ability to store solar energy in batteries is increasingly in demand. Hence, we wanted to ensure that engineers could more rapidly test their designs and reduce their time to market. Similarly, engineers working on an uninterruptible power supply can very quickly anticipate what their design will look like if they adopt an STPOWER device.
1 phase half bridgeSince STPOWER Studio supports a one-phase full bridge topology, it made sense to offer a one-phase half-bridge. This structure is common in DC-AC conversion for smaller solar inverters or motor drivers. Engineers also combine single-phase half-bridge topologies when designing a one-phase to three-phase converter. In fact, while the current version of the simulator focuses solely on DC-AC systems, we are evaluating the addition of DC-DC applications and will update this blog post with more information as they become available.
3-phase 3-level T-NPC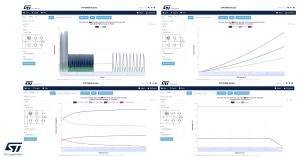
Finally, the 3-phase 3-level T-NPC (T-type Neutral Point Clamped) is increasingly popular because it improves overall efficiency by reducing switching losses thanks to a mechanism that clamps the input voltage at its halfway point. Consequently, only half of the input voltage is applied to each switch, which reduces switching losses. This creative approach greatly benefits high-power systems, such as photovoltaic inverters, power factor inverters, or motor drivers, while ensuring the overall design remains relatively small.
The post STPOWER Studio: 3 new topologies for accurate electro-thermal simulation on STPOWER devices appeared first on ELE Times.
Infineon launches new generation of GaN power discretes with superior efficiency and power density
Infineon Technologies AG has announced the launch of a new family of high-voltage discretes, the CoolGaN Transistors 650 V G5, further strengthening its Gallium Nitride (GaN) portfolio. Target applications for this new product family range from consumer and industrial switched-mode power supply (SMPS) such as USB-C adapters and chargers, lighting, TV, data center, and telecom rectifiers to renewable energy and motor drives in home appliances.
The latest CoolGaN generation is designed as a drop-in replacement for the CoolGaN Transistors 600 V G1, enabling rapid redesign of existing platforms. The new devices provide improved figures of merit to ensure competitive switching performance in focus applications. Compared to key competitors and previous product families from Infineon, the CoolGaN Transistors 650 V G5 offer up to 50 percent lower energy stored in the output capacitance (Eoss), up to 60 percent improved drain-source charge (Qoss) and up to 60 percent lower gate charge (Qg). Combined, these features result in excellent efficiencies in both hard- and soft-switching applications. This leads to a significant reduction in power loss compared to traditional silicon technology, ranging from 20 to 60 percent depending on the specific use case.
These benefits allow the devices to operate at high frequencies with minimal power loss, resulting in superior power density. The CoolGaN Transitors 650 V G5 enable SMPS applications to be smaller and lighter or to increase the output power range in a given form factor.
The new high-voltage transistor product family offers a wide range of RDS(on) package combinations. Ten RDS(on) classes are available in various SMD packages, such as ThinPAK 5×6, DFN 8×8 , TOLL and TOLT. All products are manufactured on high-performance 8-inch production lines in Villach (Austria) and Kulim (Malaysia). In the future, CoolGaN will transition to 12-inch production. This will enable Infineon to further expand its CoolGaN capacity and ensure a robust supply chain in the GaN power market, which is expected to reach $2 billion by 2029, according to Yole Group.
A demo featuring the CoolGaN Transistors 650 V G5 will be showcased at electronica 2024 in Munich from November 12 to 15 (hall C3, booth 502).
The post Infineon launches new generation of GaN power discretes with superior efficiency and power density appeared first on ELE Times.
Vishay Intertechnology IGBT and MOSFET Drivers in Stretched SO-6 Package Enable Compact Designs, Fast Switching, and High Voltages
Devices Combine High Peak Output Currents to 4 A With High Operating
Temperatures to +125 °C and Low Propagation Delay of 200 ns
Vishay Intertechnology, Inc. has introduced two new IGBT and MOSFET drivers in the compact, high isolation stretched SO-6 package. Delivering high peak output currents of 3 A and 4 A, respectively, the Vishay Semiconductors VOFD341A and VOFD343A offer high operating temperatures to +125 °C and low propagation delay of 200 ns maximum.
Consisting of an AlGaAs LED optically coupled to an integrated circuit with a power output stage, the optocouplers released today are intended for solar inverters and microinverters; AC and brushless DC industrial motor control inverters; and inverter stages for AC/DC conversion in UPS. The devices are ideally suited for directly driving IGBTs with ratings up to 1200 V / 100 A.
The high operating temperature of the VOFD341A and VOFD343A provides a higher temperature safety margin for more compact designs, while their high peak output current allows for faster switching by eliminating the need for an additional driver stage. The devices’ low propagation delay minimizes switching losses while facilitating more precise PWM regulation.
The optocouplers’ high isolation package enables high working voltages up to 1.140 V, which allows for high voltage inverter stages while still maintaining enough voltage safety margin. The RoHS-compliant devices offer high noise immunity of 50 kV/µs, which prevents fail functions in fast switching power stages.
The post Vishay Intertechnology IGBT and MOSFET Drivers in Stretched SO-6 Package Enable Compact Designs, Fast Switching, and High Voltages appeared first on ELE Times.
CoolSiC Schottky diode 2000 V enables higher efficiency and design simplification in DC link systems up to 1500 VDC
Many industrial applications today are transitioning to higher power levels with minimized power losses, which can be achieved through increased DC link voltage. Infineon Technologies AG addresses this challenge by introducing the CoolSiC Schottky diode 2000 V G5, the first discrete silicon carbide diode on the market with a breakdown voltage of 2000 V. The product family is suitable for applications with DC link voltages up to 1500 VDC and offers current ratings from 10 to 80 A. This makes it ideal for higher DC link voltage applications such as in solar and EV charging applications.
The product family comes in a TO-247PLUS-4-HCC package, with 14 mm creepage and 5.4 mm clearance distance. This, together with a current rating of up to 80 A, enables a significantly higher power density. It allows developers to achieve higher power levels in their applications with only half the component count of 1200 V solutions. This simplifies the overall design and enables a smooth transition from multi-level topologies to 2-level topologies.
In addition, the CoolSiC Schottky diode 2000V G5 utilizes the .XT interconnection technology that leads to significantly lower thermal resistance and impedance, enabling better heat management. Furthermore, the robustness against humidity has been demonstrated in HV-H3TRB reliability tests. The diodes exhibit neither reverse recovery current nor forward recovery and feature a low forward voltage, ensuring enhanced system performance.
The 2000 V diode family is a perfect match for the CoolSiC MOSFETs 2000 V in the TO-247Plus-4 HCC package that Infineon introduced in spring 2024. The CoolSiC diodes 2000 V portfolio will be extended by offering them in the TO-247-2 package, which will be available in December 2024. A matching gate driver portfolio is also available for the CoolSiC MOSFETs 2000 V.
The post CoolSiC Schottky diode 2000 V enables higher efficiency and design simplification in DC link systems up to 1500 VDC appeared first on ELE Times.
Microchip Expands 64-bit Portfolio with High-Performance, Post-Quantum Security-Enabled PIC64HX Microprocessors
The RISC-V-based MPUs support mission-critical intelligent edge applications with TSN Ethernet switching and AI capabilities
The global edge computing market is expected to grow by more than 30 percent in the next five years, serving mission-critical applications in the aerospace, defense, military, industrial and medical sectors. To meet this increasing demand for reliable, embedded solutions for mixed-criticality systems, Microchip Technology has announced the PIC64HX family of microprocessors (MPUs). Unlike traditional MPUs, the PIC64HX is purpose-built to address the unique demands of intelligent edge designs.
The latest in Microchip’s 64-bit portfolio, the PIC64HX is a high-performance, multicore 64-bit RISC-V MPU capable of advanced Artificial Intelligence and Machine Learning (AI/ML) processing and designed with integrated Time-Sensitive Networking (TSN) Ethernet connectivity and post-quantum-enabled, defense-grade security.
PIC64HX MPUs are specifically designed to deliver comprehensive fault tolerance, resiliency, scalability and power efficiency.
“The PIC64HX MPU is truly groundbreaking in the number of advanced features we are able to provide with a single solution,” said Maher Fahmi, corporate vice president of Microchip’s communications business unit. “And, integrating TSN Ethernet switching into the MPU helps developers bring standards-based networking connectivity and compute together to simplify system designs, reduce system costs and accelerate time to market.”
The integrated Ethernet switch includes a TSN feature set with support for important emerging standards: IEEE P802.1DP TSN for Aerospace Onboard Ethernet Communications, IEEE P802.1DG TSN Profile for Automotive In-Vehicle Ethernet Communications and IEEE/IEC 60802 TSN Profile for Industrial Automation.
Eight 64-bit RISC-V CPU cores—SiFive Intelligence X280—with vector extensions help enable
high-performance compute for mixed-criticality systems, virtualization and vector processing to accelerate AI workloads. The PIC64HX MPU allows system developers to deploy the cores in multiple ways to enable SMP, AMP or dual-core lockstep operations. WorldGuard hardware architecture support is provided to enable hardware-based isolation and partitioning.
“Next-generation aircraft require a new generation of processors for mission-critical applications such as flight control, cockpit display, cabin networking and engine control. The OHPERA Consortium views RISC-V technology as an essential component of the future of safe and sustainable aircraft,” said Christophe Vlacich, OHPERA technical Leader. The OHPERA Consortium is composed of leading aerospace companies with the mutual goal of evaluating new technologies for next-generation aircraft. “We are pleased to see the upcoming availability of commercial products like Microchip’s PIC64HX MPU with the compute performance, partitioning, connectivity and security needed to shape the future of aviation.”
The expected arrival of quantum computers poses an existential threat as it will make current security measures ineffective. As a result, government agencies and enterprises worldwide are beginning to call for the inclusion of post-quantum cryptography in any critical infrastructure. Addressing current and future security needs, the PIC64HX is one of the first MPUs on the market to support comprehensive defense-grade security including the recently NIST-standardized FIPS 203 (ML-KEM) and FIPS 204 (ML-DSA) post-quantum cryptographic algorithms.
The PIC64HX MPU is a powerful and versatile solution for intelligent edge applications, addressing key requirements for low latency, security, reliability and compliance with industry standards.
Development Tools
The PIC64HX MPU is supported by a comprehensive package of tools, libraries, drivers and boot firmware. Multiple open-source, commercial and real-time operating systems are supported including Linux and RTEMS, as well as hypervisors such as Xen. PIC64HX MPUs leverage Microchip’s extensive Mi-V ecosystem of tools and design resources to support its RISC-V initiatives. To help reduce development cycles and accelerate time to market, Microchip offers the Curiosity Ultra+ PIC64HX evaluation kit and is partnering with single-board computer partners.
“Aries Embedded has long been a supporter of the RISC-V ecosystem,” said Andreas Widder, Aries Embedded CEO. “We are proud to be a lead System-on-Module partner for the PIC64HX and look forward to helping Microchip enable mission critical intelligent edge applications.”
The post Microchip Expands 64-bit Portfolio with High-Performance, Post-Quantum Security-Enabled PIC64HX Microprocessors appeared first on ELE Times.
Texas Instruments expands internal manufacturing for gallium nitride (GaN) semiconductors, quadrupling capacity
Using the most advanced GaN manufacturing technology available today, Aizu, Japan, is now the company’s second factory to produce a complete portfolio of GaN-based power semiconductors
NEWS HIGHLIGHTS:
- TI adds GaN manufacturing in Japan, quadrupling its internal GaN manufacturing capacity between its factories in the United States and Japan.
- TI’s GaN-based semiconductors are in production and available now.
- TI enables the most energy-efficient, reliable and power-dense end products with the widest portfolio of integrated GaN-based power semiconductors.
- TI has successfully piloted the development of GaN manufacturing on 300mm wafers.
Texas Instruments has announced it has begun production of gallium nitride (GaN)-based power semiconductors at its factory in Aizu, Japan. Coupled with its existing GaN manufacturing in Dallas, Texas, TI will now internally manufacture four times more GaN-based power semiconductors, as Aizu ramps to production.
“Building on more than a decade of expertise in GaN chip design and manufacturing, we have successfully qualified our 200mm GaN technology – the most scalable and cost-competitive way to manufacture GaN today – to start mass production in Aizu,” said Mohammad Yunus, TI’s senior vice president of Technology and Manufacturing. “This milestone enables us to manufacture more of our GaN chips internally as we grow our internal manufacturing to more than 95% by 2030, while also sourcing from multiple TI locations, ensuring a reliable supply of our entire GaN portfolio of high-power, energy-efficient semiconductors.”
The power of GaN technology
An alternative to silicon, GaN is a semiconductor material that offers benefits in energy-efficiency, switching speed, power solution size and weight, overall system cost, and performance under high temperatures and high-voltage conditions. GaN chips provide more power density, or power in smaller spaces, enabling applications such as power adapters for laptops and mobile phones, or smaller, more energy-efficient motors for heating and air conditioning systems and home appliances.
Today, TI offers the widest portfolio of integrated GaN-based power semiconductors, ranging from low- to high-voltage, to enable the most energy-efficient, reliable and power-dense electronics.
“With GaN, TI can deliver more power, more efficiently in a compact space, which is the primary market need driving innovation for many of our customers,” said Kannan Soundarapandian, vice president of High-Voltage Power at TI. “As designers of systems such as server power, solar energy generation and AC/DC adapters face challenges to reduce power consumption and enhance energy efficiency, they are increasingly demanding a reliable supply of TI’s high-performance GaN-based chips. TI’s product portfolio of integrated GaN power stages enables customers to achieve higher power density, improved ease of use and lower system cost.”
Further, with the company’s proprietary GaN-on-silicon process, more than 80 million hours of reliability testing, and integrated protection features, TI GaN chips are designed to keep high-voltage systems safe.
Most advanced GaN manufacturing technology available today
Using the most advanced equipment available for GaN chip manufacturing today, TI’s new capacity enables increased product performance and manufacturing process efficiency, as well as a cost advantage.
Also, the more advanced, efficient tools used in TI’s expanded GaN manufacturing can produce smaller chips, packing even more power. This design innovation can be manufactured using less water, energy and raw materials, and end products that use GaN chips enjoy these same environmental benefits.
Scaled for future advances
The performance benefits of TI’s added GaN manufacturing also enable the company to scale its GaN chips to higher voltages, starting with 900V and increasing to higher voltages over time, furthering power-efficiency and size innovations for applications like robotics, renewable energy and server power supplies.
In addition, TI’s expanded investment includes a successful pilot earlier this year for development of GaN manufacturing processes on 300mm wafers. Further, TI’s expanded GaN manufacturing processes are fully transferable to 300mm technology, positioning the company to readily scale to customer needs and move to 300mm in the future.
Committed to responsible, sustainable manufacturing
Expanding supply and innovation in GaN technology is the latest example of TI’s commitment to responsible, sustainable manufacturing. TI has committed to use 100% renewable electricity in its U.S. operations by 2027, and worldwide by 2030.
The post Texas Instruments expands internal manufacturing for gallium nitride (GaN) semiconductors, quadrupling capacity appeared first on ELE Times.
Littelfuse Unique KSC DCT Tactile Switches Provide Dual-Circuit Technology with SPDT Functionality, Superior Safety
Delivers SMT and IP67 for high-efficiency performance in automotive, consumer, medical, and industrial applications
Littelfuse, Inc., an industrial technology manufacturing company empowering a sustainable, connected, and safer world, announces the C&K Switches KSC DCT Series Tactile Switches. The KSC DCT (Dual Circuit Technology) series are sealed IP67-rated, momentary-action tactile switches for surface-mount technology (SMT), designed to give users a positive, adaptable tactile feeling.
The KSC DCT is the first tactile switch to offer single pole double throw (SPDT) functionality in a small 6.2 x 6.2 x 5.2 millimeters size. The switches feature a soft actuator that withstands an operating force of 4.75N (+/-1.25N) and has a lifespan of 300K life cycles. The KSC DCT Series is a logical choice for seamless integration into existing or next-generation designs, providing outstanding, long-term reliability in even the dustiest environments.
The KSC DCT Tactile Switches offer these key features and benefits:
- SPDT Functionality: Added reliability due to one input and two outputs in a single tactile switch.
- Compact Footprint: Fits applications where space is limited.
- IP67 Sealing: Provides high contact reliability, protecting against dust and water ingress.
- J-Bend SMT Package: Enables standard assembly mounting using typical pick-and-place machines. (Gullwing-type terminations are also available.)
- Cost-Efficient: SMT makes it ideal for high-volume applications.
The KSC DCT Series Tactile Switches are ideally suited for:
- Transportation: Automotive door handles, EV charging station units, two- and three-wheelers.
- Consumer Electronics: Power tools, lawn mowers, snow blowers, home appliances.
- Medical Devices: Electrosurgical instruments, portable medical devices.
- Industrial Applications: Elevators, fire alarm equipment.
“By designing the unique functionality of dual-circuit technology tactile switches into such a small form factor, Littelfuse engineers are enabling electronics designers to provide their end-customers a journey to a safer world,” said Jeremy Hebras, Vice President of Digital & Technical Developments, Electronics Business Unit Engineering, at Littelfuse. “Thanks to the KSC DCT’s patented design, everyone can now precisely monitor the actual status of an electromechanical surface-mount technology tactile switch, even when the switch is not in use, which helps discriminate a silent signal versus a device malfunction.”
The increased safety needs of today’s end users require active failure verification: with the added single pole double throw (SPDT) functionality, the KSC DCT tactile switch allows a system to actively verify failures, like an unwanted door opening.
How it works: Dual-Circuit Technology (DCT) is a feature that creates two independent output signals inside the body of one tactile switch–Single Pole Double Throw (SPDT). The KSC DCT tactile switch has a Common, Normally Close (NC), and Normally Open (NO) pin. Suppose the designer chooses to use both NC and NO circuitry. In that case, it provides a Changeover signal that allows the designer to use both circuitries to define the logic of the signal and take actions based on the defined logic. In an automotive door handle, for example, both NC and NO circuitries are used before making any decision. The logic is at rest; the NC contact is closed while the NO contact is open. When a user pushes the button, nothing will happen until the NC contact is open and the NO contact is closed.
The post Littelfuse Unique KSC DCT Tactile Switches Provide Dual-Circuit Technology with SPDT Functionality, Superior Safety appeared first on ELE Times.
New flagship AWG cards generate waveforms with 10 GS/s speed and 2.5 GHz bandwidth
Spectrum Instrumentation launches flagship series of AWG cards in PCIe format
Scientists and engineers now have a way to produce high-frequency arbitrary waveforms, with high purity and low distortion, directly from their PC. Using the new PCIe flagship AWG cards from Spectrum Instrumentation and cost-effective COTS (Commercial-of-the-shelf) PC-parts, it is possible to generate nearly any waveform with up to 10 GS/s output rates, 2.5 GHz bandwidth, and 16-bit vertical resolution. The new cards make a powerful alternative to benchtop AWGs that often face a bottleneck when loading data for new waveforms. The cards offer a massive onboard memory of up to 8 GigaSamples (16 GB) and the possibility to stream data at up to 10 GigaBytes per second directly from CPUs or even GPUs. Four different models make up the M5i.63xx AWG series, offering a perfect fit solution for every application.
Insert the cards into a suitable PC and it turns into one of the most powerful signal generation instruments on the market. The four AWG variants deliver waveform generation with bandwidths of 2.5 and 1.5 Gigahertz (GHz) and output rates of 10, 5 or 3.2 Gigasamples per second (GS/s). The units combine 16-bit vertical resolution with programmable full-scale outputs. Single outputs deliver up to ±500 mV into 50 Ohm and ±1.0 V into high impedance loads – or double the range in differential mode.
Ultrafast data streaming
Each card comes with 2 GSample of onboard memory (8 GSample optional) and high-speed data transfer using a 16 lane, Gen 3, PCIe bus. This ultrafast bus allows data to be sent to cards at a staggering 10 GB/s. For demanding applications, data can even be continuously streamed directly to the AWG for replay in a FIFO mode – a process that allows almost limitless waveform production. Add Spectrum’s SCAPP driver package, which allows FIFO streaming directly to and from a GPU, and turbocharge the waveform processing even further.
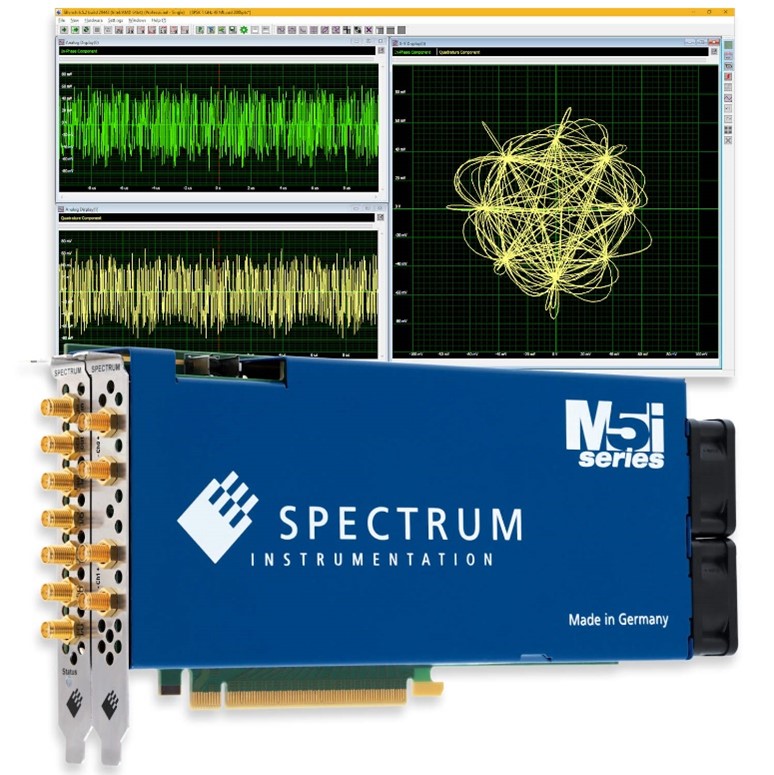
Versatile Waveform Generation
Waveforms can be output in Single-shot, Repeated and Multiple Replay modes. To maximize memory efficiency, Multiple Replay can be used to output segmented data and can also be combined with FIFO streaming. Waveform replay can be initiated by a simple software command or via a trigger event. Trigger signals can be input on two external trigger lines.
Multi-channel Systems
Individual cards have one or two analog output channels. To create larger multi-channel systems, cards can be connected together using the company’s proprietary Star-Hub clock and trigger synchronization module. Star-Hub allows systems with up to eight cards to share a common clock and trigger, delivering fully synchronous output rates of 5 GS/s on up to 16 channels, or 10 GS/s on up to eight channels.
Mixed AWG and Digitizer Systems
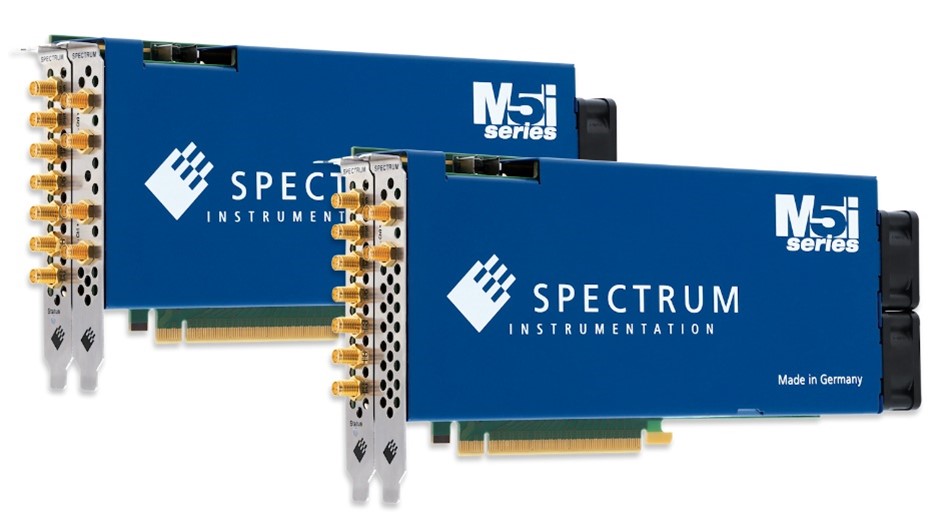
The four new models of the M5i.63xx AWG series and the seven variants of the M5i.33xx digitizer series are designed for working together, making them ideal for use in stimulus-response, receiver-transmitter or closed-loop type testing systems. For example, if two Star-Hubs are used, ultrafast MIMO systems can be built that contain up to 8 AWGs and 8 digitizers. This allows the creation of systems with up to 16 transmit and 16 receiver channels, each channel with 5 GS/s.
Easy connection with other devices
For easy system integration, the front-panel hosts four multi-function SMA connectors. These can perform a variety of Input/Output tasks like Asynchronous Digital-I/O, Synchronous Digital-Out, Trigger Output, Run and Arm status flags, or the System Clock. By switching the multi-function I/O lines to digital outputs, another four synchronous output channels can be added to the AWG. As such, a single AWG card can generate up to two analog and four digital outputs, in parallel, at full speed. As an option, a Digital Pulse Generator firmware is available to turn the four digital outputs into digital generators outputs. All these features are very helpful when interfacing with other equipment for experiment control or in OEM projects.
Fully programmable
Fully programmable, the cards run under Windows or LINUX operating systems, using today’s most popular and powerful software languages. All products are shipped together with SDKs for C++, C#, Python, VB.NET, Julia, Java and IVI. Drivers are also provided for third-party software products LabVIEW and MATLAB.
Five Year Warranty
The Spectrum M5i.63xx series AWGs are available for immediate delivery. All cards are shipped factory tested and include a five-year warranty, with software and firmware updates, free of charge, for the lifetime of the product.
The post New flagship AWG cards generate waveforms with 10 GS/s speed and 2.5 GHz bandwidth appeared first on ELE Times.