Українською
  In English
Новини світу мікро- та наноелектроніки
Meta’s New Large-Language Model to Run on Intel and Qualcomm Hardware
Finally decided to continue a project I started 6 months ago
![]() | submitted by /u/Blytical [link] [comments] |
A Guide to Effective Probe Selection for Low Noise Power Integrity Measurements
Universal analog-to-digital multiplexer-demultiplexer

The possibility of creating a universal analog-to-digital multiplexer-demultiplexer is shown.
Wow the engineering world with your unique design: Design Ideas Submission Guide
As is known, a multiplexer and a demultiplexer is an electronic device designed for selective signal transmission from one of several inputs to one output, or, on the contrary, signal transmission from one input to one of several outputs. Channel switching is performed by digital signals supplied to the control inputs.
First, let’s consider the operation of a control node containing key elements controlled by digital input signals. Figure 1 shows an example of such a node based on discrete elements such as bipolar or field-effect transistors; or using two “NOT” logic elements. When applying to the input (Inp) of such a node, signals of the level “Log. 1»/«Log. 0” the output signal levels Out1 and Out2 are switched. To switch, for example, four channels, it is necessary to use two similar control nodes.
Figure 1 Control node using discrete elements such as bipolar or field-effect transistors.
Figure 2 shows the electrical circuit of the signal transmission channel switching unit, made using two control units, Figure 1, as well as diode-resistive elements. The signals from the ABCD outputs are sent to the corresponding ABCD control inputs of analog switches, Figure 2.
Figure 2 Electrical diagram of the analog switch control unit using discrete elements.
When digital signals of the level “Log. 1” or “Log. 0” are applied to the inputs, X1 and/or X2 of the control nodes implements four combinations of selective appearance at the outputs of the ABCD levels “Log. 1”. The inputs and outputs of analog switches have the property of reversibility, which allows the device to be used both as a multiplexer and a demultiplexer.
Figure 3 shows a variant of the control unit for analog switches from a set of logic elements “NOT” and “2AND”.
Figures 3 Electrical diagram of the analog switch control unit using logic elements.
To be able to disable the passage of any signals from the input to the output of the device, or vice versa, the scheme shown in Figure 2 can be supplemented with the function of general disconnection of the passage of signals, Figure 4. When an Inhibit signal of the “Log. 1” level is applied to the input, the transistor Q opens and shunts the control inputs of the analog switches ABCD through the diodes.
Figures 4 Electrical diagram of the device for general disconnection of signals passing through all switching channels.
Figure 5 shows the possible pin arrangement of the universal analog multiplexer/demultiplexer chip, its schematic representation, and truth table.
Figure 5 Possible pin layout of the universal analog multiplexer/demultiplexer chip, its schematic representation, and the truth table.
Figure 6 demonstrates the possibility of using such a device as a multiplexer when signals from 4 sources are fed to the ABCD inputs. When digital control signals are applied to inputs X1 and X2, one of the signals taken from sources E1–E4 will pass to the output Y of the device.
Figure 6 Using a universal analog multiplexer/demultiplexer as a multiplexer, its graphical designation, equivalent circuit, and truth table.
Figure 7 shows the options for using a universal analog of a universal multiplexer/demultiplexer as a demultiplexer.
Figure 7 Using a universal analog multiplexer/demultiplexer as a demultiplexer, its graphical designation, equivalent circuit, and truth table.
Figure 8 shows an example of using a device for selectively enabling/disabling information transmission channels from sources E1–E4 to the outputs/inputs of ABCD.
Figure 8 Examples of using a universal analog multiplexer/demultiplexer to control the passage of signals through one of the channels involved.
The described device can be used for switching both analog and digital signals of positive polarity, however, with a slight improvement of the device, it can be converted to switch signals of both positive and negative polarity.
Michael A. Shustov is a doctor of technical sciences, candidate of chemical sciences and the author of over 800 printed works in the field of electronics, chemistry, physics, geology, medicine, and history.
Related Content
- Rectangle and triangle waveform function generator
- Simple XOR logic elements on transistors
- Universal purpose optoelectronic logic elements
- Universal logic element on one transistor and its applications
The post Universal analog-to-digital multiplexer-demultiplexer appeared first on EDN.
Rohde & Schwarz and IPG Automotive unveil a complete Hardware-in-the-Loop automotive radar test solution
Rohde & Schwarz has teamed up with IPG Automotive, a pioneer in virtual test driving, to redefine automotive radar Hardware-in-the-Loop (HIL) integration testing thereby reducing the cost by bringing Autonomous Driving (AD) testing from the proving ground to the development lab. Combining the CarMaker simulation software from IPG Automotive with the R&S AREG800A radar object simulator and the R&S QAT100 advanced antenna array provides vehicle manufacturers with the ability to simulate ADAS/AD scenarios like those defined in the Euro NCAP in a controlled, safe, time-efficient and cost-reducing way. This combination provides automotive OEMs and radar sensor suppliers with a comprehensive radar sensor testing platform. It is applicable for validation and real-time, closed-loop characterization of radar sensors and enables compliance with Association for ASAM standards.
IPG Automotive’s CarMaker simulation solution is designed for the development and end-to-end testing of cars and light commercial vehicles at all development stages from MIL/SIL to HIL and VIL. The open integration and test platform allows vehicle manufacturers, engineering services companies and Tier 1 suppliers to implement virtual test scenarios in the application areas of powertrain, vehicle dynamic chassis functions and ADAS/AD functions. Thanks to a high-resolution 3D engine, the simulation of the complete sensor stack is possible in very high detail. Seamless integration into existing tool landscapes is also possible with a variety of supported standards and interfaces like the ASAM Open Standards.
The R&S AREG800A, in conjunction with the R&S QAT100, generates multiple artificial objects, each with independent range, Radar Cross Section (RCS), angle (azimuth/elevation) and radial velocity. The Open Simulation Interface (ASAM OSI) links R&S AREG800A with the CarMaker HIL test automation, creating a comprehensive test setup, proving extremely valuable for realistic driving scenario assessment within radar HIL testing. Moreover, the solution includes a Scenario Editor, specifically designed for non-simulation experts. This feature greatly simplifies the process of testing maneuver-based driving scenarios and their associated complex traffic-related configurations and makes it accessible to a wider range of professionals.
The simulation solution CarMaker features an advanced Test Manager, capable of building and running automated test sequences. It can run tests individually or simultaneously, locally or in the cloud, providing flexibility and scalability. Additionally, it automates the calculation and evaluation of Key Performance Indicators (KPIs) and generates reports automatically, thereby saving considerable time and effort.
The Model Manager CarMaker helps to define the configuration of virtual vehicle prototypes. This enhances the realism and accuracy of simulations, making them more useful for testing and development.
Andreas Höfer, Chief Technology Officer at IPG Automotive: “With the collaboration between Rohde & Schwarz and IPG Automotive, we intend to provide the automotive industry with a comprehensive Hardware-in-the-Loop integration test solution, bringing tests from the proving ground into the lab, resulting in significant time and cost savings in the development process.”
The post Rohde & Schwarz and IPG Automotive unveil a complete Hardware-in-the-Loop automotive radar test solution appeared first on ELE Times.
STMicroelectronics reveals high-performance, state-of-the-art wireless microcontrollers ready for incoming cyber-protection regulations
Highly integrated chips support multiple wireless technologies and the latest security standards, perfectly addressing requirements for smart industrial, medical, and consumer devices
STMicroelectronics, a global semiconductor leader serving customers across the spectrum of electronics applications, has revealed the next generation of its short-range wireless microcontrollers. These innovative, all-in-one components enable wearables and smart objects including smart home devices, health monitors, and smart appliances to become ever more miniaturized, easy to use, secure and affordable.
Short-range wireless technologies like Bluetooth LE, Zigbee and Thread (popular in smart meters and smart buildings) are the fabric connecting smart devices to home bridges, gateways, and controllers including smartphones. As we all seek solutions for making life more economical, sustainable, and comfortable, vendors are looking to bring creative and high-performing new solutions to market more quickly, within tight cost constraints. They need to be stylish, too: tiny, low-profile, or even embedded out of sight in other equipment, such as in smart bulbs. Going wireless is a part of this trend, for freedom, flexibility, and fashion.
Wireless microcontrollers like ST’s new STM32WBA5 product line allow a one-chip solution that’s extremely compact, reduces the bill of materials, and shortens the time to market by relieving wireless design challenges. Also, being compatible with the development tools and software packs of the STM32 microcontroller development ecosystem, the new line simplifies migrating existing products designed for wired connections.
The new series’ flagship STM32WBA55 microcontroller can communicate using multiple wireless standards concurrently, including low-energy Bluetooth LE 5.4, Zigbee, Thread, and Matter (Thread RCP). Matter border router is a perfect match with the STM32WBA5 for this new open-source connectivity standard for smart-home and IoT (Internet of Things) devices. In this way, the STM32WBA55 supports a great user experience while simplifying hardware and software engineers’ development journey, aiding affordability and time to market for the new product.
With this new generation, ST has also introduced support for the recently completed Bluetooth LE Audio specifications that enable exciting and innovative new products for richer listening and hearing experiences. These include the new Bluetooth Auracast feature, which opens the door to a new world of audio broadcasting applications.
“Lead customers are already appreciating the enhanced wireless performance, flexibility, and security of our latest STM32WBA wireless microcontrollers. They are creating diverse products including smart thermostats, tracking devices, smart chargers, headsets, power tools, and smart meters,” said Benoit Rodrigues, Wireless MCU Division General Manager, STMicroelectronics. “The extensive software ecosystem that provides communication stacks, microcontroller-specific software packs, sample code, and tools helps developers bring new products to market based on these MCUs quickly and efficiently.”
The STM32WBA series is the first wireless MCU in the market to achieve the important SESIP (Security Evaluation Standard for IoT Platforms) Level 3 security certification. With this, smart devices containing STM32WBA microcontrollers are ready to satisfy the US Cyber Trust Mark and EU Radio Equipment Directive (RED) regulations due to become mandatory in 2025.
“We work with smart asset tracking devices which are connected through Bluetooth to mobiles and through mobile apps to the cloud, enabling us to deliver complete asset tracking management for our customers. ST’s microcontrollers bring wireless connectivity to our multi-mode trackers,” said Olivier Hersent, CEO, Abeeway (Actility Group). “We have selected the new wireless connectivity product, STM32WBA5, for its enhanced performance with ultra-low power radio capabilities, which is key for our battery-powered devices. They ensure stable connectivity in the harsh industrial environment where we operate, combined with security that meets the highest industry standards.”
“We believe that gaming peripherals should be as unique and accessible as the gaming community itself,” said Tom Roberts, CTO, Performance Designed Products (PDP). “ST and PDP have worked together through several Video Game generations, and we have used STM8 and STM32 devices for many years. ST products consistently provide the features we need in our highly competitive market segment. We recently selected ST’s short-range wireless connectivity product, the STM32WBA5, based on the integrated MCU and Bluetooth low-energy technology, as the right solution for a new, groundbreaking game controller. The STM32WBA offered us an ideal combination of performance, peripherals, cost efficiency and ecosystem support that enabled simple and fast development.”
Sample requests and pricing information are available from local ST sales offices. ST will introduce a ready-to-use module containing the STM32WBA, integrated with necessary external components including power-supply and antenna-balancing circuitry, in June 2024.
The post STMicroelectronics reveals high-performance, state-of-the-art wireless microcontrollers ready for incoming cyber-protection regulations appeared first on ELE Times.
IQE’s annual revenue falls 31.2% in 2023, impacted by excess inventory
5 considerations when choosing a haptics manufacturer

In the rapidly emerging world of haptic technology, businesses are increasingly recognizing the value of integrating haptic feedback into their products. In a study conducted by Immersion Corp. on consumer attitudes about high-definition haptic effects in smartphones, a vast majority of respondents (~90%) said they prefer mobile phones that offer haptic feedback. Another study from the University of Oxford found that the participants performed tasks better when notifications came with haptic feedback.
This shows that haptics is poised to play a big role in shaping user perceptions and behaviors. Market research firm CB Insights projects that the haptics industry is projected to grow at a CAGR of 3.7%, reaching a value of $ 5 billion by 2028. Moreover, a gradual shift toward touch-centric consumer gadgets is paving the way for more opportunities in haptic technology.
Source: Titan Haptics
Integrating haptics into your next product comes with a plethora of benefits. It not only enhances the user experience, leading to improved brand image and customer satisfaction, but can also boost accessibility, safety, and precision. Additionally, haptics can enable businesses to offer customization options, future-proof their offerings, and explore new revenue streams.
That said, the manufacturing of haptic products is no small feat. It requires a blend of multidisciplinary expertise, careful consideration of user experience, seamless integration of technology, meticulous material selection, and strategic planning for scalability.
What are the issues to think about when manufacturing haptic products?
- Multidisciplinary expertise
Haptic technology is a complex field that requires a deep understanding of electronics, firmware, software, and mechanical engineering. For small- to medium-sized enterprises (SMEs), this multidisciplinary nature can pose a significant challenge.
Consider a startup that specializes in virtual reality (VR) gaming and wants to incorporate haptic technology into its products to enhance user experience. This startup may have a team of software engineers who are proficient in developing VR games but lack the expertise in haptic technology.
In order to incorporate their desired haptics, a partnership with a full-service manufacturer or product development firm may be beneficial. This can help bridge the necessary expertise in electronics, firmware, software, and mechanical engineering. As a result, the startup can focus on its core competency, while ensuring that its products are enhanced with the latest haptic technology.
- User experience (UX)
The user experience (UX) should be a priority when designing haptic products. The haptic feedback should be instinctive and enhance the user’s engagement with the product. However, the transition from prototype to mass production may introduce changes that could impact the intended user experience.
This is where a manufacturer with expertise in manufacturing haptic products becomes invaluable. Manufacturers can recommend vibration damping to significantly enhance the haptic feedback. They can also procure high-quality components like buttons—loose buttons that create noise during haptic feedback can negatively impact the user experience.
Manufacturers can also tailor the product to its intended use. For instance, if the product is designed for use in a heavy-duty industry, the motors need to be sealed against environmental factors. This ensures they are not compromised by dust and debris, thereby maintaining the quality of the haptic feedback and, in turn, the user experience.
- Material selection
The choice of materials in the production of haptic products can significantly influence the quality of the haptic feedback. Selecting the appropriate materials is a vital part of manufacturing haptic products, as an unsuitable choice can negatively impact the product’s functionality and effectiveness.
For example, using a rigid material for a haptic glove, which needs high touch sensitivity and responsiveness, may not deliver the subtle haptic feedback required for an immersive virtual reality gaming or medical training experience.
The importance of material selection goes beyond the product’s performance. It also impacts user comfort, market attractiveness, and, ultimately, sales. Experienced haptic product manufacturers can provide insight and suggest suitable materials for the product’s intended use. They can also assist companies in making educated decisions regarding material selection, which also has an impact on cost.
- Scalability
In addition to the technical considerations, it’s also important to consider the scalability of a product. This involves consideration of manufacturing processes, cost, and quality control measures. A well-thought-out design should be scalable for mass production without compromising on quality.
Scalability in manufacturing is a crucial factor in effectively boosting production volumes to satisfy rising market needs while keeping or even reducing costs per unit. Imagine a startup that has created a new high-definition haptic gaming controller. At first, production is on a small scale, with only a few hundred units produced each month. However, as the product becomes more popular, demand surges.
With this increased demand, there are additional factors to consider. While producing more units could decrease the cost per unit due to economies of scale, it could also inflate total costs if not properly managed.
Therefore, it’s crucial to collaborate with a trusted manufacturer to ensure that the quality of the product aligns with the company’s standards, values, and customer expectations.
- Importance of IP protection
In the tech-driven haptic industry, intellectual property (IP) is a critical asset. Protecting this asset can be a complex and challenging process, particularly when dealing with international suppliers. A robust IP protection plan is critical in providing peace of mind for a business.
Consider a company that has developed a unique haptic feedback mechanism for a wearable. This mechanism could be a huge differentiating factor that sets the product apart from similar wearable devices. However, without a solid IP protection plan, the company risks losing its competitive edge if the mechanism is copied or reverse-engineered by a rival.
This risk is particularly high when dealing with international suppliers, as different countries have different laws and regulations regarding intellectual property. For example, if the company is sourcing components from China, it needs to be aware of the Chinese laws on intellectual property and ensure that its IP protection plan is robust enough to protect its technology.
Why a qualified manufacturing partner matters
Manufacturing haptic products can be incredibly challenging and requires careful consideration of the factors outlined above. A qualified manufacturing partner should be capable of addressing all these factors and offering advice on how haptic products can be optimized for their use case.
By understanding these complexities and partnering with a reliable manufacturing program, businesses can successfully navigate the haptic industry and create products that deliver value to their customers.
Kyle Skippon is head of engineering at Titan Haptics Inc.
Related Content
- Implement haptics in touch-based user interfaces
- Eat, Spend, Run: Students Write Next Chapter of Haptics
- Surface haptics technology enriches touchscreen interactions
- Enhancing your touch screen design with haptic tactile feedback
- Can You Feel It? Advanced Haptics Enhances the User Experience
The post 5 considerations when choosing a haptics manufacturer appeared first on EDN.
Rambus Presents First GDDR7 Memory Controller in the Age of AI 2.0
Class-D Amplifiers 60 Years Apart (1964/10W, 2024/500W)
![]() | submitted by /u/jellzey [link] [comments] |
Common-Mode Noise in Differential Transmissions: Characteristics and Causes
Transphorm and Weltrend add 150mΩ and 480mΩ 650V integrated GaN system-in-packages
Vishay selects Aixtron’s G10-SiC multi-wafer batch technology
Silicon Labs Plunges Into Energy Harvesting With New Wireless SoCs
Sidereal time versus solar time

About time keeping, the definition of “sidereal” is “of or with respect to the distant stars (i.e. the constellations or the fixed stars, not the sun or planets)”, as defined after a google search. With that in mind, a quick and admittedly simplistic look at keeping earthly time is shown in Figure 1.
Figure 1 A comparison between solar days of 24 hours and sidereal days of 23 hours, 56 minutes, and 4.091 seconds.
As the earth moves around the sun in its yearly orbit, the absolute direction in which some fixed point on the earth’s surface that is aimed directly at the sun changes. We who sit on the earth at some particular place, maybe in our backyards, see the sun reach its peak in the sky once every twenty-four hours and we call that a solar day. Disregarding leap years, leap seconds, and the like; our wristwatches, tabletop alarm clocks, and other timepieces keep track of our personal time on a solar day basis.
Measured with respect to absolute space, however, the solar day requires an earth rotation per “day” of slightly more than 360°. Thus, when we take the universe at large as some fixed entity, with respect to that entity, the earth completes an exact 360° rotation in an average time of only 23 Hours, 56 Minutes, 4.091 Seconds which is a shorter time span.
That shorter time span is a sidereal day which differs from and is slightly less than a solar day.
Have you checked your watch lately?
John Dunn is an electronics consultant, and a graduate of The Polytechnic Institute of Brooklyn (BSEE) and of New York University (MSEE).
Related Content
- Calculate the dBm yielded by a neutron star collision
- A test guide for small-satellite constellations and NewSpace applications
- How It Was: You used to be able to see the stars at night
- Twinkle twinkle little stars!
The post Sidereal time versus solar time appeared first on EDN.
Rohde & Schwarz introduces new R&S SMB100B microwave signal generator for analog signal generation up to 40 GHz
Available with four frequency options each covering from 8 kHz to 12.75 GHz, 20 GHz, 31.8 GHz or 40 GHz, the new R&S SMB100B microwave signal generator from Rohde & Schwarz brings excellent output power, spectral purity, very low close-in phase noise, and practically no wideband noise to analog microwave signal generation.
The new R&S SMB100B analog microwave signal generator from Rohde & Schwarz offers outstanding, market-leading performance for analog signal generation up to 40 GHz in the midrange class. Thanks to its easy operation and comprehensive functionality, the versatile R&S SMB100B is now the first choice for all applications requiring clean analog signals or high output power from 8 kHz to 40 GHz. Typical applications include testing radar receivers, semiconductor components, upconverters, downconverters or amplifiers. Thehigh output power and low phase noise make it ideal as a source for simulating interferers for blocking tests.
The R&S SMB100B microwave signal generator features a signal purity which combines very low single sideband (SSB) phase noise, excellent non-harmonics suppression, and low wideband noise for all carrier frequencies. For users seeking even better close-in phase noise and frequency stability, and less temperature-based variation in performance, in addition to the standard OXCO reference oscillator, a higher performance version is available for all frequency ranges. In addition to the conventional 10 MHz reference frequency, users can choose optionally 1 MHz to 100 MHz as well as 1 GHz reference frequency signals. Optional high output power of measured 25 dBm at 20 GHz and 19.5 dBm at 40 GHz is activated by keycode, so users can install it at any time. Considering the microwave frequency range covered by the instrument, the R&S SMB100B microwave signal generator is light (10.7 kg) and compact, fitting in a 19” rack and only two rack units in height.
The level accuracy of the output directly from an R&S SMB100B itself is excellent. With each increase in the frequency of the required signal, the challenge of obtaining the correct level input to a device increases: The R&S SMB100B supports two additional features to compensate for path losses and variations in the signal caused by setups with additional test fixtures, cables or amplifiers. These features help to provide the wanted power level at the reference plane i.e. at the input of the device under test. One of them, the user correction function (UCOR), compensates if the frequency response of the setup is known and stable. However, there are still unknown factors especially if the setup includes additional active devices such as an amplifier. Then 1/2 the frequency response of the setup with an external additional amplifier can vary over level or temperature. Closed-loop power control can compensate for all these variations by continuously measuring the input level to the DUT i.e. at the wanted reference plane with a suitable R&S NRP power sensor feeding its measured level back to the generator to adjust the output power accordingly. More details about this use case can be found in the application note 1GP141.
The R&S SMB100B is user-friendly in every detail. Users can create their own customized menus, so that the parameters they most use are always available. They can generate code to automate measurements first made manually with the SCPI macro recorder while the measurements are set up and run, then use the code generator to export the instructions in languages such as MATLAB. Thanks to R&S Legacy Pro, the R&S SMB100B (and other Rohde & Schwarz test equipment) can be used to emulate other instruments such as R&S SMB100A or competitor instruments directly, as a drop-in replacement using the existing code.
The new R&S SMB100B microwave signal generator up to 40 GHz is now available from Rohde & Schwarz and expands the R&S SMB100B analog signal generator family with its established RF models up to 6 GHz.
The post Rohde & Schwarz introduces new R&S SMB100B microwave signal generator for analog signal generation up to 40 GHz appeared first on ELE Times.
Tata Elxsi is Improving Aircraft Manufacturing Performance Through its Industry 4.0 solutions
Having delivered 13% revenue growth in FY24, Tata Elxsi is among the world’s leading design and technology service providers across industries including Automotive, Broadcast, Communications, Healthcare, and Transportation. They mark high competence in servicing through design thinking and development in digital technologies like IoT, Cloud, Mobility, Virtual Reality, and AI.
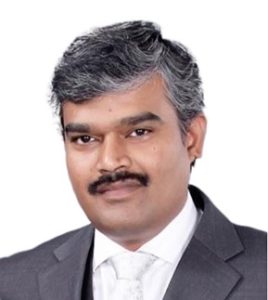
Rashi Bajpai, Sub-Editor at ELE Times spoke with Jayaraj Rajapandian, Head of Avionics, Transportation, Tata Elxsi on various aspects of aerospace/ aviation – from what’s trending to what the future holds for the industry.
This is an excerpt from the interaction.
ELE Times: What are some of the latest trends in Aerospace electrification?
Jayaraj Rajapandian: The aerospace industry is rapidly evolving, with many innovations redefining the field. One of the latest advancements is in aerospace electrification. This is a major boost to meet the UN sustainable development goals set for the Aerospace industry. It involves implementing electric propulsion technologies such as electric motors and turbo-electric propulsion by using electric energy to power the aircraft fully or in hybrid mode.
The electrification of propulsion systems made Urban Air Mobility (UAM) vehicles a reality and it is a step closer to commercial operation. Smaller aircraft and drones provide decreased emissions, quieter operation, and improved efficiency. Electrical actuators drive fuel efficiency and are replacing the hydraulic-driven actuators.
Hybrid-electric propulsion systems combine traditional fuel-powered engines with electric propulsion systems. The larger aircraft adapts them to increase fuel efficiency and reduce emissions.
Sustainable Aviation Fuels (SAFs) can be used to reduce aviation’s environmental impact. However, the investment to scale the SAF production is to be monitored against aviation demands.
Electric Vertical Takeoff and Landing (eVTOL) vehicles enable vertical takeoff and landing, which reduces the dependency on infrastructure like a dedicated runway. Vertiport unlocks the potential for urban air mobility. These vehicles will be incorporated more for logistics and aerial combat vehicles.
Moreover, advancements in battery technologies are seeing exponential growth in fuel cells for storage, effective power conversion, and distribution, necessitating effective battery management solutions. Lithium polymer batteries enable long-endurance owing to their lower weight and higher power storage.
ELE Times: Give us some insights into the future innovations in Unmanned Air Systems.
Jayaraj Rajapandian: Unmanned Air Systems (UAS) have been incredibly useful in improving efficiency, reducing costs, reaching remote and inaccessible areas, improving defence systems, and, most importantly, enhancing safety. The primary focus is to improve autonomous navigation and control by incorporating cutting-edge technologies such as Artificial Intelligence (AI) and Machine Learning (ML) to operate effectively in complex environments and all-weather conditions and push the endurance for extended missions.
Currently, commercial usage of UAVs is being monitored by regulators, mainly when operating beyond the visual line of sight (BVLOS). However, advanced sensor and payload technologies such as LiDAR and thermal imaging systems could help improve the availability and reliability.
Unmanned Combat Systems consist of aerial, land, and underwater drones. Unmanned aerial vehicles are used to gather intelligence, conduct surveillance, and reconnaissance (ISR), and carry munitions. Governments worldwide recognize Unmanned Combat Systems as an asset comparable to manned fighter jets as they consume a significant portion of defence budgets. Researchers are exploring swarm intelligence to enable several drones to work together and operate collectively, ensuring the mission is never compromised, even if many UAVs are lost.
ELE Times: Elaborate on some of the latest technologies in avionics development for advanced navigation and control.
Jayaraj Rajapandian: In the past couple of decades, satellite-based navigation and communications systems have become more widespread, electronic systems have become more scalable, and higher redundancy has become more common in recent aircrafts. Fly-by-wire flight control systems have replaced mechanical controls with electronic interfaces, allowing for precise and adaptive control of aircraft flight surfaces. Using a single pane of glass in the cockpit flight decks turned the operation to be more seamless. However, by the mid-2030s, ICAO predicts that airspace will witness double the current traffic and the industry requires more than incremental innovation, a transformation is needed.
Currently, the focus is on bringing compact form factors and platforming the systems. Aerospace OEMs and technology partners are collaborating on this next journey. RISC-V-based processing units are gaining attention for their security features and custom-built capabilities that meet OEMs’ needs. The collaboration on Avionics such as FMS, used in different aircraft supplied by various vendors, to create a unified family of products signifies a strategic move towards standardization and interoperability in the aviation industry. This reduces inventory costs for OEMs and the training costs of airlines.
Innovations to counter deceived sensors to manage spoofing, anti-jamming and to distinguish friends from foes, and security in communications are gaining attention. A growing number of regional players are developing Avionics for UAVs, breaking the technology entry barrier. To stay competitive and relevant, defense OEMs require transformational effort to reduce cycle time which typically takes 5 to 7 years. Digital Twin, Investment in Big-Data processing with High Processing Computing capability can accelerate this cycle time.
Tata Elxsi’s advanced process flow can be used in the development of a cloud-based Digital Twin of a sub-system. The features developed from the Digital Twin are scalable and can be used for multiple systems simultaneously.
ELE Times: How can AI/ML be adopted for aerospace design and maintenance?
Jayaraj Rajapandian: With AI and ML capabilities, aerospace design and maintenance can improve efficiency, reduce downtime, and improve the health of systems. AI and ML can analyze extensive datasets from simulations, past designs, and real-world operations to pinpoint the most effective configurations for aircraft components, structures, and systems.
AI and ML tools also help to build virtual prototyping and testing of aircraft systems and components. It generates precise simulations using high-processing computers, anticipating performance traits, and fine-tuning design parameters. More importantly, AI and ML algorithms also help in predictive maintenance. These algorithms can analyze sensor data from aircraft systems and components to detect anomalies, predict failures, and schedule maintenance proactively. AI and ML tools also help in care for health monitoring systems and analysis of root causes. We will soon see certifications through simulations of numerous scenarios exercised on the system models.
Our solution accelerator for TEDAX- Tata Elxsi’s big data platform is being used to build system models and visualize the data. Tata Elxsi’s AI-based Video Analytics AIVA resolves complex scenarios in real-time.
ELE Times: How is Tata Elxsi enhancing aircraft production efficiency?
Jayaraj Rajapandian: The aerospace industry is picking up the pace in demand post-slowdown due to the COVID-19 pandemic. With the boost in demand, OEMs will need to enhance their production efficiency by tapping into advanced manufacturing technologies, adding cost-effective suppliers, and integrating product lifecycle management techniques. Technologies like 3D printing, robotics, digital twins, and automated assembly systems can enhance aircraft production.
Tata Elxsi designs and implements Industry 4.0 solutions, improving manufacturing performance. We are also working with OEMs in identifying suppliers to source raw materials, build-to-spec, and certify their products.
The post Tata Elxsi is Improving Aircraft Manufacturing Performance Through its Industry 4.0 solutions appeared first on ELE Times.
Rio Tinto awards Missouri S&T $875,000 to research recovering gallium and germanium from copper refining waste
It's Wednesday, so here's my small apartment workbench. Moving to a new place soon and I hope I get more space.
![]() | submitted by /u/OftenDisappointed [link] [comments] |
A faulty memory IC caused Voyager 1 to send incoherent messages. After 5 months, scientists figured out how to work around it and restore communications.
![]() | submitted by /u/1Davide [link] [comments] |
Сторінки
